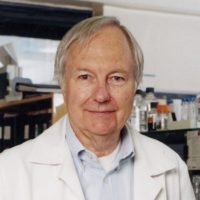
James E. Darnell Jr.
The Rockefeller University
For an exceptional career in biomedical science during which he opened two fields in biology —RNA processing and cytokine signaling — and fostered the development of many creative scientists.
The 2002 Lasker Special Achievement Award honors an imaginative scientist, influential textbook writer, and esteemed educator — James Darnell, Jr. of The Rockefeller University. For 45 years, he has led breakthroughs in our understanding of gene regulation and has fostered the careers of more than 125 scientists, many of whom have gone on to make creative contributions through their own work. By opening up two fields of biology — RNA processing and cytokine signaling — Darnell has expanded our knowledge about the means by which nucleated cells model their immature RNAs into useable forms and reprogram their genes in response to physiological signals from their surroundings.
Darnell’s monumental achievements and insights have influenced every arena of animal biology, and have opened avenues toward therapies for medical conditions that include anemia and cancer. While making these advances, he has supervised two generations of students and postdoctoral fellows. In addition to the scientists he has mentored in his own lab, he has co-written two popular textbooks that have taught tens of thousands more. His influence extends beyond that exerted by his research and writing activities: He continues to play a leading role in revitalizing The Rockefeller University by recruiting superb junior faculty to that institution and cultivating their scientific development.
As scientists were marveling at the elegant simplicity of this bacterial system, Darnell was tracking RNA inside mammalian cells. Soon he made a surprising finding: Very long RNAs gradually disappear from the nucleus, where they are made, and shorter RNAs show up in the cytoplasm. The nucleotide composition of the large and the smaller molecules resemble each other and are characteristic of RNAs known to compose ribosomes, the protein-making factories of the cell. Together, the results indicated that mammalian cells pare down their original RNAs; they release several mature forms of ribosomal RNA (rRNA) from a giant precursor molecule. Darnell coined the term “RNA processing” to describe this phenomenon in a 1963 paper that reports this work.
When Darnell analyzed the largest and most fleeting RNA in the nucleus, he discovered that its composition does not resemble that of rRNA, but instead corresponds to that of total mammalian DNA and mRNA. He called this RNA heterogeneous nuclear RNA (hnRNA) and speculated that hnRNA is the nuclear precursor to mRNA just as pre-rRNA is the precursor to rRNA. Perhaps the mRNA lay in the middle or at the ends of the hnRNA molecule, awaiting liberation.
He and Mary Edmonds, of the University of Pittsburgh, independently found that some hnRNAs and almost all mRNAs carry a string of adenine nucleotides — a poly(A) — at one end. Furthermore, Darnell showed that poly(A) appears first in hnRNA and later in cytoplasmic mRNA, supporting the notion that the first molecule gives rise to the second one. Subsequently, scientists discovered a distinguishing chemical structure called a cap on the other end of both mRNA and hnRNA. Darnell found that many hnRNA molecules and all mRNA molecules carry one poly(A) tail and one cap. These observations posed an enigma: How could a long hnRNA with a tail and cap give rise to a short mRNA with the same characteristic tags at its ends? In retrospect, the answer is obvious, but at the time, the dogma that DNA sequence always corresponded exactly to mRNA sequence — and that genes were thus uninterrupted — forced scientists to surmount a tremendous intellectual barrier before stumbling upon the solution in 1977. Two teams — led by Phillip Sharp of MIT and Richard Roberts of Cold Spring Harbor Laboratory — vaulted this hurdle when they used electron microscopy to look at DNA bound to the mRNA it encodes. Large regions of the DNA looped out, and the groups proposed that internal spans of sequence — now called introns — were removed as hnRNA was transformed into mRNA. With this discovery, the field of mRNA splicing burst onto the scientific scene.
Knowing how mRNA was formed, Darnell next decided to back up a step and explore how cells regulate its production — or transcription — from DNA, and how they activate particular sets of genes to accomplish specialized physiological tasks. By the early 1980s, he had discovered that a liver cell could remain a liver cell only when it resided in its natural milieu. When he separated cells from each other, they stopped manufacturing liver-specific mRNAs within a few hours, although they continued to produce basic housekeeping mRNAs. Without constant signals from their normal habitat, liver cells lost the mRNA profile that helps give them their identity.
These observations made Darnell wonder how cues from outside the cell could spark specific gene activity. He suspected that he could analyze this issue by studying how proteins called interferons (IFNs) instigate human cells to guard themselves against viruses. These proteins — subdivided into groups called alpha, beta, and gamma — belong to a hormone family called cytokines that rev up antimicrobial defenses. Immediately after binding to the outside of a cell, IFNs spur the manufacture of proteins that quash viral duplication and render the infected cells more susceptible to attack by the immune system.
To get a handle on the cellular players that turn on genes in response to IFN, Darnell decided to work backwards, reasoning that the genes triggered by the cytokine would lead him to their molecular inducers. To find IFN-prodded genes, he identified mRNAs that accumulate in response to IFN-beta. His and another research group showed that the mRNA hike didn’t depend on new protein synthesis, implying that the cell possessed the IFN-responsive gene activator — or transcription factor — in a dormant state before stimulation.
After isolating a short stretch of DNA that lies next to IFN-alpha-induced genes, he sought cellular machinery that could sit down on this sequence and drive transcription of adjacent test genes. This strategy produced three nuclear factors that bound to that particular piece of DNA and not others; one of them appeared specifically in IFN-treated cells. This IFN-responsive transcription factor was what he sought; it hangs out in the cytoplasm, waiting for IFN to jolt it into action. Two thousand liters of IFN-treated cell cultures later, Darnell’s students and postdoctoral fellows had purified this factor, which consisted of four proteins. These were among the first purified proteins that respond to signals from outside the cell by binding to DNA and stimulating genes. Darnell subsequently named two of them STAT1 and STAT2, echoing the “STAT” call of a hospital loudspeaker — in part because they trigger genes quickly.
Once he had isolated the proteins that provoke the genes, he wondered what the cell uses to sense IFN outside the cell and goad the Stats into action. He soon discovered that when IFN attaches to its receptor on the surface of cells, STAT1 and STAT2 acquire a chemical group — phosphate — known to animate proteins. Other teams identified the enzymes that tack on these phosphates as Janus kinases, or JAKs, which cozy up to molecules that span the cell’s membrane. When IFN binds on the outside, these molecules prompt the JAKs to modify Stats. A new signaling system — the Jak-Stat pathway — was born, and provided the first detailed account of a pathway that leads from a tickle at the cell’s surface to changes in gene activity inside its nucleus. The findings elucidated how cells can dramatically switch physiological directions in response to environmental signals.
Since its discovery, the mammalian Stat family has expanded to seven members, and its relatives have popped up in a wide range of organisms, including worms, slime molds, and flies. IFN-alpha represents just one chemical prod for these transcription factors and the physiological consequences of Stat activation extend beyond the anti-viral response. STAT3, for example, participates in animal cell growth regulation, inflammation, resistance to cell death, and early embryonic development in response to a variety of stimuli — and drugs that act on this Stat might hold therapeutic value. Darnell showed that a hyperactive version of STAT3 spurs rampant cellular reproduction in culture and that an inhibitory version blocks this unrestrained growth. These results and others, together with the observation that more than 50 percent of human cancers contain constantly active STAT3, suggest that the protein normally prevents deranged cancer cells from committing suicide. Thus, a pharmaceutical agent that thwarts STAT3 might repel cancer. Other Stats, too, participate in medically relevant pathways. A drug that incites STAT5 (recombinant erythropoietin) is already on the market to combat anemia.
In addition to discovering the fields of RNA processing and Stat signaling, Darnell has co-written two textbooks — General Virology and Molecular Cell Biology—that have instructed countless students. He has personally influenced a tremendous number of trainees over the course of his career, having mentored more than two dozen PhD students and 100 postdoctoral fellows in his lab. These individuals include an exceptional number of creative scientists, including Sheldon Penman, David Baltimore, Jonathan Warner, Ronald Evans, Joseph Nevins, and Jeffrey Friedman.
Darnell has also played an indispensable role in building a strong independent junior faculty at The Rockefeller University. A long-held and cherished tradition of The Rockefeller University ordained that early career investigators should work in senior faculty members’ labs. In 1985, Darnell co-chaired a university committee charged with finding strong young candidates, and hiring them as independent scientists. The first five researchers refused the university’s offers because they didn’t believe that they’d be allowed the autonomy they desired. Now, owing in large part to Darnell’s effective leadership, Rockefeller competes for junior faculty with other top-notch institutions. The program has engaged several dozen young scientists in the last decade, 20 of whom have earned tenure. Scientists at other institutions have hailed this innovation, saying that it has greatly broadened the research fabric of the university.
For almost half a century, Darnell has had a major impact on science worldwide. He has uncovered major themes in RNA’s function as an informational molecule, and provided a molecular explanation for how the cell heeds signals from its environment. Throughout his career, he has devoted himself to nurturing young scientists, assuring his legacy through their achievements as well as his own.
by Evelyn Strauss
Award presentation by Eric Kandel
For the past 50 years — the past half-century — out of which emerged the coherent molecular-based biology that we now enjoy, the scientific community has been fortunate in having been served brilliantly by several truly outstanding leaders. Each of these combined scientific creativity with a broad vision and a commitment to biology as a whole. James Darnell, whom we honor today with a Lasker Special Achievement Award, is one of these great leaders. More than anyone else, Jim helped redirect molecular biology from its origins in the study of genes in bacteria and bacterial viruses to a focus on the larger picture, the genes in the cells of higher organisms. Moreover, he led by example, by making not one but two fundamental contributions. One, in the 1960s he discovered that, in mammalian cells, messenger RNA, which carries the message from the DNA template on how to make proteins, is processed from a larger to a smaller functional form. Two, in the 1980s he discovered how signals outside the cell lead to the turning on of genes inside the cell.
Like Faulkner, Jim proved remarkably creative. At the beginning of his career in the mid-1950s, the major experimental system being used in molecular biology was the bacterium E. coli. But Darnell realized while he was still in Harry Eagles’ lab at the NIH that biology was becoming sufficiently mature to take on the much more difficult study of more complex cells — mammalian cells — and the viruses that inhabit them. Jim’s work was pivotal in moving the focus of modern biology from bacterial to animal cells. He was among the first to appreciate that the cells from higher animals could be cultured and studied much like simple bacterial cells. Much as Max Delbrück and Luria had earlier used viruses that inhabit bacteria to creatively probe genetic mechanisms in bacteria, Darnell now sought to use animal viruses to define the mechanisms whereby mammalian cells make proteins.
Unlike the situation in bacteria, little was known in 1961 about how information encoded in the template DNA in higher animal cells was transferred to proteins. In bacterial cells, the idea was first entertained that the amino acids, the building blocks of protein, were lined up directly on the template of DNA, an idea that was soon dismissed. Next, some thought that the ribosomes, the multimolecular machinery that synthesizes the proteins might serve as the template carrier of the information from the DNA in the nucleus to the cytoplasm. This idea was also soon rejected. Finally, in 1961, Sidney Brenner and François Jacob discovered a special short-lived species of RNA in bacteria, which they called “messenger RNA,” which delivered information from the DNA template to the ribosomes. Darnell therefore spent the year 1961 with Jacob in Paris. When Darnell returned to the United States to join the faculty at MIT, he set out to find the equivalent ‘messenger’ in animal cells and to determine the rules that control its production. In a series of papers in the early 1960s, Darnell helped establish the first link in animal cells between RNA, the protein synthesis machinery, and its final products.
His overreaching ideas were forged through a detailed analysis of another kind of RNA, called ribosomal RNA, an RNA that actually forms part of the ribosomes, the machinery where the proteins of the cells are synthesized. Here, Jim made the remarkable discovery that the size of the ribosomal RNA molecule ‘shortened’ during its journey from the nucleus, where it was made, to the cytoplasm, where it was used. This provided the first clear evidence that information in the genome is copied into an RNA precursor that is ‘processed’ to a smaller, functional product.
Darnell now honed in on messenger RNA. In 1971 he solidified the fundamental principle that for messenger RNA as well, there is a large precursor and small product, by finding that the larger precursor RNA population in the nucleus and the messenger RNA population in the cytoplasm shared a common signature: They both had the same tail.
In focusing on the precise relationship of the genome template to the initial RNA copy, Darnell turned to a human-specific adenovirus, a virus that causes cold symptoms, and wrote prophetically, “the adenovirus system offers many advantages as a model for the study of possible processing of nuclear RNA into mRNA because viral sequences can be specifically identified by DNA-RNA hybridization.” By using a single gene of adenovirus, the late gene, Darnell was able to show that here again the large primary transcript is processed into its smaller final form within a few minutes of its synthesis.
These fundamental studies, in a set of papers that stand as models of scientific rigor and transparency, set the stage for one of the major discoveries in modern biology: the discovery that the genes of higher organisms differ dramatically from bacterial and viral genes in having interruptions in their instructions for what protein to make. Thus, genes of higher organisms are not continuous like those of bacteria but are split into pieces consisting of protein coding and non-coding regions. The processing of the larger RNA precursors into the smaller messengers involves the removal of these non-coding regions, by a mechanism called splicing. The coding regions are then stitched together to obtain the final instructions for protein synthesis. The discovery of splicing was made by two groups, one led by Phil Sharp at MIT and the other by Richard Roberts at Cold Spring Harbor, using the same adenovirus RNA pioneered by Jim Darnell. Both Sharp and Roberts have emphasized repeatedly that they would not have carried out their experiments were it not for the adenovirus system and the framework of thinking about mRNA processing developed in Darnell’s earlier work. The Sharp-Roberts experiments, in turn, provided us with a radically new understanding of the discontinuous organization of genes in higher organisms and therefore in our genome — a radical departure of what we had learned as dogma from bacterial geneticists. So Jim was right to get us to insist that studying elementary process in higher organisms would introduce new principles.
In the 1980s, Darnell opened up and largely elucidated, on his own, a second field of biology. In studying the response of cells to viruses, he discovered a new mechanism for a cell’s reaction to events outside the cell. This mechanism does not use diffusible small signaling molecules inside the cell; instead the signal is carried directly from the membrane, where events on the outside are perceived, to the nucleus by a protein that directly activates genes.
Darnell approached this problem by studying the interferon response pathways. The interferons are hormones that are released following viral infection and which produce proteins that make cells resistant to viral infection. In studying the interferon response, Darnell discovered a new class of proteins that can serve as transcription factors, or gene activators, that link events at the cell surface directly to the turning on of genes. To emphasize their dual role, Darnell called these factors STATs (for signal transducers and activators of transcription).
Darnell discovered that in the uninfected, unstimulated normal cell, these transcription factors were tethered to receptors for interferon at the cell membrane. Upon binding interferon, this receptor pairs with proteins called JAK kinases, identified by George Stark and Ian Kerr, which activate the STATs. The activated STAT transcription factors then pair with each other and move into the nucleus, where they turn on genes.
Since each type of interferon turns on different genes, Darnell next asked: how is this specificity achieved? He discovered that the STATs constitute a family of proteins and that different interferons recruit different STATs to mediate their actions. Thus, Darnell’s work provided an elegant solution to how the different interferon protein sensors at the cell surface can lead directly to the selective turning on of genes and of a unique cellular response. Indeed, after Darnell’s discovery of the actions of interferons, many other important factors involved in a myriad of biological processes were found to act through this general pathway (including the interleukins IL-2, IL-4, IL-6, granulocyte macrophage colony-stimulating factor, erythropoietin, growth hormone, prolactin, and leptin).
What is so remarkable about Darnell’s career is that he has combined this sustained high level of scholarship and influence on biology with an extraordinary sense of pedagogy and academic leadership.
In 1986 Darnell co-authored, with Harvey Lodish and David Baltimore, the first edition of Molecular Cell Biology. Together with Molecular Biology of the Cell, by Alberts, Raff, and their colleagues, the Darnell textbook helped define for many undergraduate and graduate students the modern molecular cell biology that we know and love. These two textbooks have been extremely influential. They have not only documented the remarkable unification that biology has achieved on the molecular level, but they also illustrated the power, generality, and elegance of biological principles that have emerged in the last 50 years, a power that now places biology on the same level of pedagogy as physics and chemistry.
Finally, Darnell has trained in his lab a cadre of major scientists, including David Baltimore, currently president of Caltech, and a number of superb molecular biologists: Sheldon Penman, Jonathan Warner, Ron Evans, Joe Nevins, Ed Ziff, and Jeff Friedman, and indirectly, by example, his talented son Robert Darnell. His interest in helping start young scientists on the path to an independent career led to his efforts to restructure Rockefeller University. Darnell realized early on that the future of Rockefeller University would be dependent on its ability to attract and develop a strong, young faculty. This had been hampered by an outdated system where junior faculty were typically not independent but were recruited from within the labs of full professors. By systematically and tactfully guiding changes in this system, Darnell made it possible in 1990 to initiate and guide the long-term effort of building a new faculty.
Darnell’s has been a remarkable career of lifetime achievement in science, pedagogy, national, and university leadership — career that has made, and continues to make, a great impact on modern biology, on the students that he has trained, on the institutions to which he has belonged and to the national enterprise which he helped lead.