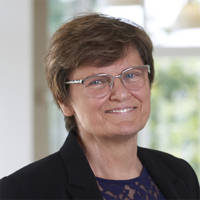
Katalin Karikó
BioNTech
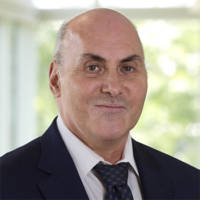
Drew Weissman
University of Pennsylvania
For the discovery of a new therapeutic technology based on the modification of messenger RNA—enabling rapid development of highly effective Covid-19 vaccines
The 2021 Lasker~DeBakey Clinical Medical Research Award honors two scientists—Katalin Karikó (BioNTech) and Drew Weissman (University of Pennsylvania)—who discovered a new therapeutic technology based on the modification of messenger RNA. This breakthrough enabled rapid development of highly effective Covid-19 vaccines. In addition to providing a tool for quelling a devastating pandemic, the innovation is fueling progress toward treatments and preventives for a range of different illnesses.
In the early 1960s, messenger RNA (mRNA) sprang into the spotlight as the agent that encodes protein, and the possibility of using this nucleic acid for medical purposes began to tickle scientists’ imaginations. mRNA could, in principle, provide a means to turn a person’s cells into factories for any desired protein. Such methodology might replenish essential substances that are in short supply or introduce microbial components as a vaccine. Furthermore, it offered numerous advantages over DNA-based strategies. Unlike DNA, mRNA would not threaten the recipient cell’s genomic integrity because it cannot integrate into the chromosome and interrupt resident genes or wreak other mutational havoc. As an undergraduate in the mid 1970s, Karikó found the promise of mRNA-directed protein replacement irresistible. Since then, she has devoured information and mastered techniques with that enterprise in mind.
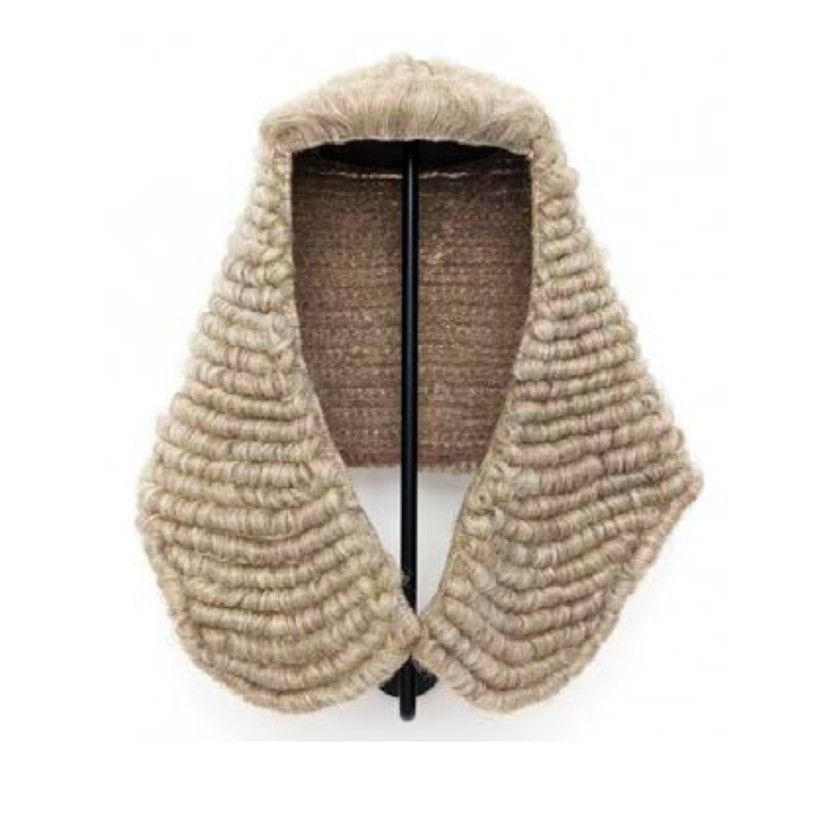
The Surprise Element: A Distinctive Characteristic of Creative Scientists, Artists, and Comedians
Truly creative works of science and art produce unexpected and surprising results—just like the punch line of a good joke that generates an unfamiliar twist on a familiar idea.
Acceptance remarks
Acceptance remarks, Katalin Karikó
I grew up in a small Hungarian town with a population of ten thousand. As a little girl I liked to climb up trees to check out the bird nests and watch the neighbor’s cow give birth. I was curious. My science teachers made sure that I stayed curious for life and encouraged me to keep learning.
Though I had never actually seen a scientist, I decided that’s what I will do. Now today receiving the Lasker award, I have been able to reflect on my humble beginnings and the long winding road on which I have arrived.
In high school my teacher handed me a book called The Stress of Life in which Hans Selye wrote that “adopting the right attitude can convert a negative stress into a positive one.” This book was my guide through the years as I continued the journey of becoming a scientist.
I remembered Selye’s words when I experienced failures–as a student, or in the lab–so I adopted the right attitude, searched for ways to improve myself, to work harder, be more creative and perform better. I always try to focus on things that I can do and not waste any time on things that I cannot.
I began my work with RNA as a graduate student in 1978 and have been passionate about this fragile molecule ever since. I learned the ways that RNA can be made and modified, how it degrades or translates into proteins.
I love the enigmatic nature of RNA-related phenomena, and enjoy revisiting experimental outcomes that I couldn’t explain decades earlier. I am always delighted when scientific puzzles that I tried to decode myself are ultimately solved by others.
My greatest joys in science have been the small and large victories in the lab. Even if an experiment fails, it teaches us something that we could build upon.
Together with my colleagues we inched towards a solution, building upon discoveries of scientists who came before us, and created the optimal RNA suitable for therapy. Never in a million years would I have imagined that it would be used to create a vaccine to combat a global pandemic.
While I am accepting my Lasker Award, I think about all those hard-working fellow scientists who are–just like me–passionate about their work and immensely contributed to the success of the Covid-19 mRNA vaccine.
I also think about all of the young girls who may become inspired by my story and want to become scientists. To them I say: stay curious, adopt the right attitude and stay on the track no matter how long and winding that road may be.
Acceptance remarks, Drew Weissman
Those who know me, know that I’m more at home in my lab than I am in the spotlight. And no offense intended, but I would much prefer to be there than in front of a camera right now…as you may be able to tell.
My wife, Mary Ellen, and children Rachel and Allison, are often frustrated by my disinterest in celebrating my own successes. When research indicated that mRNA vaccines surpassed 90 percent effectiveness at preventing Covid-19 and almost 100 percent at preventing hospitalizations from it, my family wanted to do something special. Once we were fully vaccinated, I said, “Alright, if you really want to celebrate, I guess we can go have a nice dinner.” I just wanted to get back to the lab.
I am, however, truly grateful that work I did has helped the world. That’s the hope of every physician-scientist. And I am honored to be the recipient of a Lasker Award and to be counted among a remarkable group of recipients, which includes my long-time scientific partner, Katalin Karikó.
At Penn, Dr. Kariko and I investigated mRNA as a medical intervention. We were both excited by the promising possibilities of an area of medicine we did not feel had been sufficiently explored. And after all, we were at the University of Pennsylvania, an institution of “firsts”–the nation’s first medical school and a place where breakthroughs happen.
So, we conducted experiments. Each spark of something interesting, whether a finding we expected–or even more exciting, the ones we didn’t–motivated us to continue. Together we designed and conducted experiments, often trading emails into the early hours–unable to wait until the next day to share particular findings or ideas. We just kept at it.
Although the investigations that we began over two decades ago have culminated in significant discoveries and a vaccine against a pandemic-causing virus, the work continues. I’m thrilled to say that my lab is pursuing new mRNA vaccines to guard against a host of infectious diseases, like influenza and HIV, and even a pan-coronavirus vaccine that could offer protection against all viruses in the coronavirus category.
Equally important to discovery is ensuring that everyone is able to realize the benefits. We are collaborating with researchers around the world to help them create their own mRNA vaccines for Covid-19 to increase the global supply for people in low- and middle-income countries. And we are taking opportunities to educate people on the science behind this often-misunderstood vaccine.
Scientists know the work is never over because, as much as it’s black and white when looking at data on the page, the implementation and utilization of discovery is open-ended. The work is never over because of the need for scientific breakthroughs: not just for the current pandemic, but for autoimmune diseases, neurologic diseases, and rehabilitation after trauma, and other infectious diseases. Making the discovery and answering your own curiosity is thrilling but making an impact on human beings is what excites me the most.
Today, as I take a second to appreciate this good fortune, that our work has helped millions of people around the world, I also want to recognize the scientists who are “at home” in their own labs right now, fueling the engine of progress, doing the work that may lead to something amazing. I’ll join you back home in my lab in the morning…or if I get antsy, maybe in a few hours. Together, we’ll see what the future holds.