The development of the modern cochlear implant — a breakthrough device that allows deaf and hearing-impaired people to understand speech — is a triumph of engineers and physicians. But the story involves an even larger cast of characters. Blake Wilson, co-director of the Duke Hearing Center at Duke University, remembers meeting twice a month for more than a decade spanning the 1980s with a team that included not just engineers and otolaryngologists (ear, nose, and throat doctors), but also audiologists, neuroscientists, and psychiatrists, all working to solve a problem that many experts called unsolvable: using multiple electrodes implanted deep in the ear (cochlea) to stimulate the auditory nerve and restore hearing.
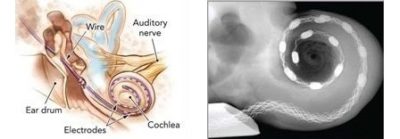
Left: Illustration of the inner ear portion of a cochlear implant. Right: X-ray of a cochlea that shows an implanted 12-electrode device, including the wires that carry the electrical signals.
Although nobody described the cochlear implant research this way at the time, it was the perfect example of convergence, a term that was coined much later, in 2011. “Initially it was regarded as an interdisciplinary effort,” says Wilson, who won the 2013 Lasker~DeBakey Clinical Medical Research Award, along with Graeme M. Clark and Ingeborg Hochmair, for his pioneering work on the modern cochlear implant. But in retrospect, Wilson says, the research represented more than an interdisciplinary collaboration. It was the integration of distinct disciplines and technologies into a “unified whole” that gave rise to new ideas, approaches, and discoveries. In essence, it was the definition of convergence, as laid out in a 2014 report by the National Academies of Sciences, Engineering, and Medicine.
Since the idea of convergence first entered the scientific conversation, “it is almost everywhere to some degree and growing in importance,” says Phillip Sharp, professor of biology at Massachusetts Institute of Technology (MIT) and one of 12 MIT researchers that penned a white paper in 2011 that introduced the term. Sharp and his co-authors referred to convergence as the “third revolution” in life sciences; it follows the first revolution of the 1950s to 1970s, when molecular and cellular biology advances flung open the door to new understanding of disease states, and the second revolution in the 1980s and 1990s that ushered in genome sequencing and unprecedented genetic insights. Although all three revolutions relied on synergy between disciplines, convergence is the broadest both in terms of the disciplines and technologies it encompasses and the potential for scientific impact, says Sharp, 1988 Lasker Basic Medical Research Laureate.
Evidence of the spread of convergence can be found in the increasing number of biologists at universities working in physics and other quantitative science departments, Sharp says. Many academic institutes, both new and retooled, are also organized around a convergence approach, he adds, including at MIT (the Koch Institute for Integrative Cancer Research, where Sharp is a professor), Harvard University (the Wyss Institute for Biologically Inspired Engineering), and the University of Chicago (Institute for Molecular Engineering).
The need for convergence springs from the vast amount of data that life sciences are amassing — from the Human Genome Project to data sets of protein interactions. In some cases, research groups started plucking pieces of data to study from these big data sets seemingly arbitrarily, Sharp says. “It was so unsatisfactory. The only way I thought the field could really move in a way that took advantage of all [the data] was to more fundamentally incorporate technology.”
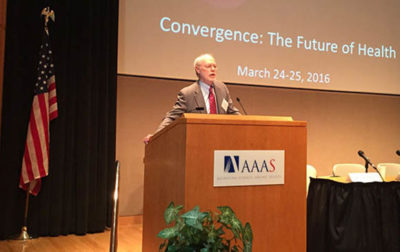
Phillip Sharp, professor of biology at MIT and Lasker Laureate, presents a 2016 MIT report on convergence science at the American Association for the Advancement of Science (AAAS).
Although taking a convergence approach could potentially benefit research endeavors in any discipline, it could especially transform health care, as a 2016 MIT report, which Sharp co-chaired, details. For example, researchers are turning to machine learning algorithms, which use data to learn patterns and make predictions, to create tools for computer-based scoring of mammography and histology images that can identify individuals at risk of breast and prostate cancer, respectively, more accurately than clinicians. This field of computer science could improve clinical decision-making in myriad areas; another possible application is using data from wearable devices to identify risk factors for heart attacks and strokes.
The growth in convergence, which kicked off less than 10 years ago, was preceded for at least a decade by another field of life science with a similar philosophy: quantitative biology. One definition of this field, embraced by some who practice it, is simply using quantitative approaches — such as mathematical, statistical, and engineering models or computer programs and algorithms — to study biological systems. Quantitative biology could mean biologists learning on their own the math or physics they need to know to advance their work. And there are still plenty of examples of the traditional model of a biology professor collaborating with or consulting, for example, a physics professor in a different department across campus, says Louis Gross, director of the National Institute for Mathematical and Biological Synthesis at the University of Tennessee Knoxville, one of the first quantitative biology centers in the country, established in 2008.
But as more and more quantitative biology centers crop up at academic institutions, researchers are setting a higher bar to meld life science and quantitative disciplines in a way that looks a lot like convergence. In one case, the new Quantitative Biology Initiative at Harvard University (which is distinct from Harvard’s Wyss Institute), aims to develop new statistical and computational methods for addressing the critical problem of identifying which data from an enormous data set are important, says Sharad Ramanathan, professor of molecular and cellular biology, stem cell and regenerative biology, and applied physics, and one of the leaders of the Initiative. Meanwhile, Carole LaBonne, professor of molecular biosciences at Northwestern University and one of the leaders of the university’s new NSF (National Science Foundation)-Simons Center for Quantitative Biology, hopes her work with mathematicians in the center — collaborations so close she calls them “embedding” — will lead to new mathematical models for explaining embryonic development.
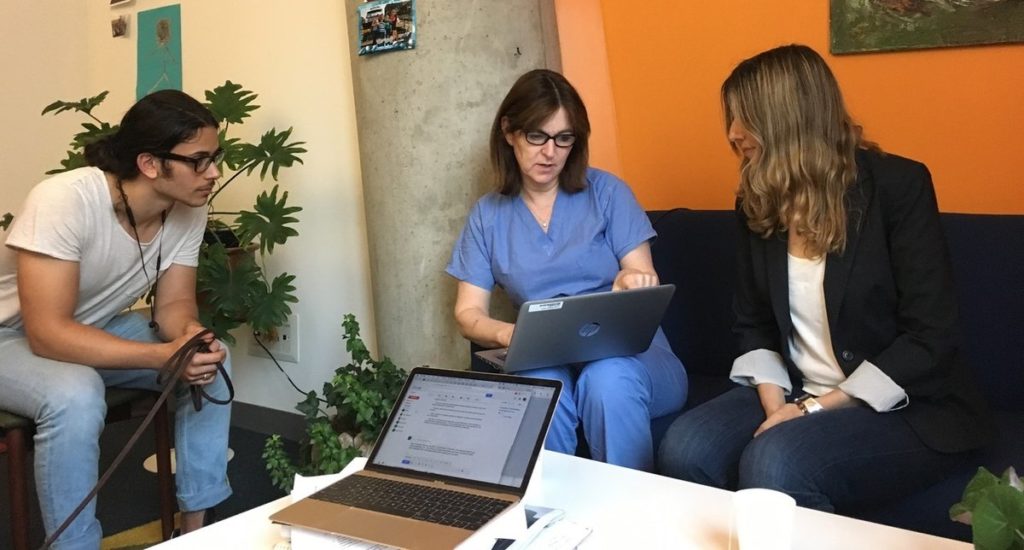
Regina Barzilay (right), professor of electrical engineering and computer science at MIT, and Constance Lehman (middle), professor of radiology at Harvard Medical School, discuss a machine learning tool they developed for assessing breast cancer risk, with MIT PhD student Adam Yala (left).
When biologists and quantitative scientists work together in close partnership, their respective areas of expertise enrich the other; such experiences are the cornerstones of convergence. “I’m as interested in integrating biology into physics and engineering as I am integrating those disciplines into biology,” Sharp says. There is a longer history of the latter scenario, he notes, with physicists and engineers heading into biology labs to contribute to solving the structure of DNA and proteins, but there is starting to be more reciprocity. While there is still the need for “the solitary creative investigator” who stays firmly planted in his or her own discipline, the world of scientific research cannot be comprised of only that type of investigator as data sets and technologies get more and more complex, Sharp says.
Pushing the concept of convergence even farther, the 2014 National Academies report on the topic called out the need to include social sciences and the humanities in the great melding of disciplines. These fields hold the power to “unlock” breakthrough technologies and help them reach their full potential for societal impact, says Joseph DeSimone, chair of the report and CEO of Carbon, a US-based technology company. Humanities could come into play, adds DeSimone, in addressing how a new medical device could be covered by insurance and exploring the business and ethical aspects of introducing it to the general population.
By Carina Storrs
This story is the first in a series about convergence and other fields under its umbrella, such as quantitative biology. The next stories will take a look at how graduate programs are training future generations of scientists in these fields and what career paths in this field look like.