The patient who arrived at the National Institutes of Health (NIH) in Bethesda, Maryland, one day in 1968 was only seven, but she was already primed for a heart attack. Her cholesterol levels were more than seven times higher than normal. So much cholesterol was coursing through her blood that the fat-like substance was visible as lumpy, orange-tinged nodules beneath her skin.
The girl suffered from a severe form of the rare genetic condition known as familial hypercholesterolemia (FH). Cholesterol levels soar in the disease, driving premature atherosclerosis that can lead to fatal heart attacks, sometimes in childhood. The girl’s condition captured the attention of Joseph Goldstein and Michael Brown, two NIH post-doctoral researchers and physicians who vowed to find out what went wrong in FH. Her case “opened up what we’ve done for the last 50 years,” says Goldstein, who is now chair of the Lasker Research Awards jury.
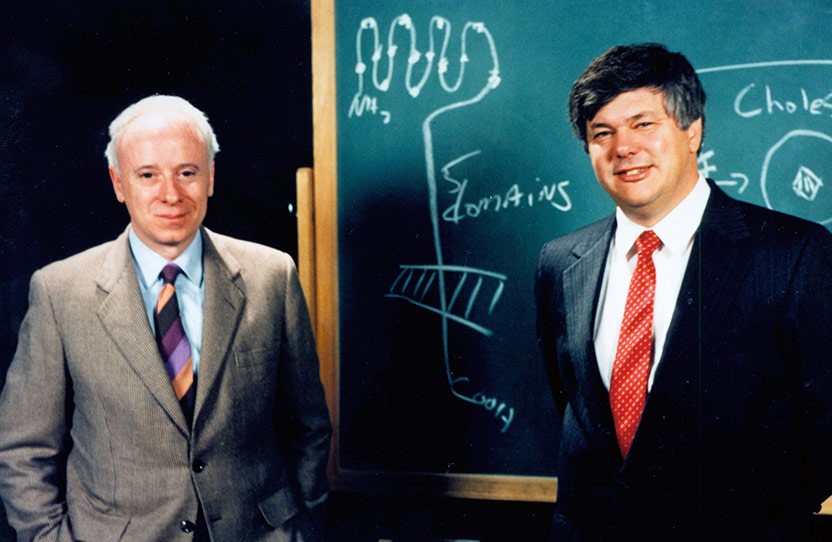
Joseph Goldstein (left) and Michael Brown in 1985, the year they won a Lasker Award and a Nobel Prize for their work on LDL metabolism.
Image courtesy of University of Texas Southwestern Medical Center
The two scientists not only cracked the mystery of how FH accelerates atherosclerosis, they also rewrote the understanding of how the body manages cholesterol and uncovered new opportunities for combating heart disease. In 1985 the pair received a Lasker Award and a Nobel Prize. Goldstein’s and Brown’s findings were “a complete revolution,” says cardiologist Christopher Cannon of Harvard Medical School.
Their work is one of several landmark Lasker-winning advances in the field of cardiovascular disease, along with the discovery of lipid-lowering statins, the synthesis of anti-hypertension drugs known as ACE inhibitors, and the development of prosthetic heart valves. “These are major scientific accomplishments that have had incredibly broad ramifications for human health,” says cardiologist Deepak L. Bhatt, also of Harvard. Statins, for instance, have become staples of heart disease prevention and are among the most prescribed drugs in the world. These advances also laid the foundation for a series of new approaches that may improve the prospects for patients with cardiovascular disease.
Receptive to LDL
Brown and Goldstein began delving into FH in 1972, after they reunited on the faculty of the University of Texas Southwestern Medical Center. At the time, researchers knew that much of the body’s cholesterol cruises through the blood inside particles of low-density lipoprotein, or LDL, and that people with high levels of LDL are prone to heart attacks. “Our goal was to find out what regulates the level of LDL in the body,” Brown says.
In just over a decade, the two researchers identified a protein crucial for that regulation, pinpointed its gene, and uncovered how certain mutations produce FH’s artery-plugging LDL levels. “If you had told us in 1972 that we’d make that much progress, says Brown, “I’d have thought you were certifiably crazy.”
One reason the scientists succeeded, however, was what Goldstein has described as a “somewhat heretical” choice — they decided to investigate the disease in cultures of skin cells. Because the liver is the hub of cholesterol metabolism in the body, most researchers insisted that the process should be studied in liver cells. However, obtaining them from patients with FH was extraordinarily difficult.
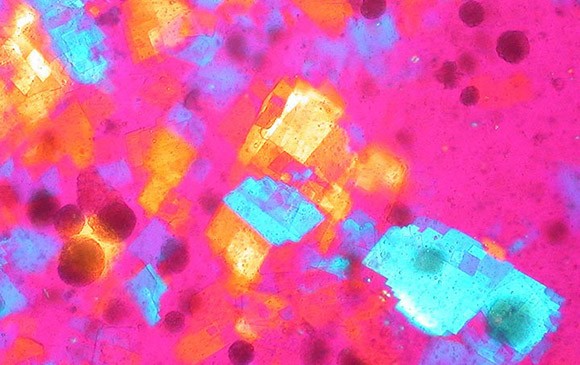
Cholesterol crystals
Image courtesy of Ed Uthman, via Wikimedia Commons
The scientists’ approach quickly provided an insight into the disease. In a 1973 experiment, the pair found that the skin cells of healthy people differ from those of patients with FH. Cells require cholesterol to maintain their membrane coat. When LDL was plentiful in culture, Brown and Goldstein found normal cells met their needs mainly by absorbing LDL. But if LDL was scarce, the cells cranked up cholesterol synthesis. Cells from patients with FH, by contrast, always manufactured large quantities of cholesterol, no matter how much LDL was available.
Goldstein and Brown thought they knew why. They surmised that one of the key enzymes for manufacturing cholesterol, HMG-CoA reductase, was faulty in patients with FH and did not respond to signals to throttle back its activity. However, they soon discovered that they were wrong. The patients had perfectly good HMG-CoA reductase. “That was a complete surprise,” says Goldstein.
A year later, the scientists’ heretical approach paid off again when they discovered the reason for the difference between normal and FH cells. Normal cells carry a receptor protein embedded in their membrane that enables them to capture and take in LDL. The receptor, which the scientists and their colleagues isolated in 1982, proved to be instrumental for controlling LDL levels in the body. When cells require more cholesterol, they make and deploy more receptor molecules, thus absorbing extra amounts of LDL and reducing its levels in the bloodstream. Because of mutations in the receptor’s gene, patients with FH produce defective copies of the protein or none at all. As a result, LDL builds up in the blood and infiltrates the walls of arteries, leading to atherosclerosis early in life.
The findings, Brown says, suggested a new way to treat or prevent atherosclerosis not only in patients with FH, but also in the general population. “We recognized that if we could decrease cholesterol in cells, that would lead to an increase in LDL receptors and a decrease in LDL in the blood.” But no one had located a drug to curb cholesterol synthesis — until the winner of a 2008 Lasker Award, the Japanese biochemist Akira Endo, came along.
Molding a Cholesterol Drug
Decades before you could follow somebody on social media, Goldstein and Brown were using a scientific literature alert service to follow Endo’s work. Little did they suspect he was about to revolutionize medicine by discovering statins. Endo grew up on a farm in northern Japan, where he became enthralled by mushrooms and other fungi. That passion served him well when he was working at the Sankyo Research Laboratories in Tokyo in 1968. Given carte blanche to choose a project, Endo decided to search for a chemical that blocked cholesterol synthesis.
Endo picked cholesterol, he wrote in a 2010 retrospective, because he had spent time studying in New York, where he noticed the “rather rich dietary habits of Americans compared to those of the Japanese,” as well as the disparity in heart attack rates between the two countries. Plenty of other scientists were chasing drugs to inhibit HMG-CoA reductase, which determines how fast the cholesterol-producing reactions progress. Endo knew that fungi were excellent chemical factories and suspected that one of them might manufacture a compound that would inhibit the enzyme.
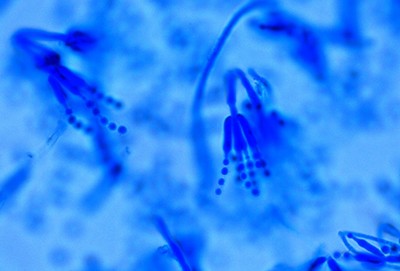
Penicillium citrinum fungus used by Endo to isolate compactin
Image courtesy of thunderhouse4-yuri blog
Confirming that suspicion took plenty of work. He and his colleagues grew and tested more than 3800 types of fungi to determine whether their chemical products stymied HMG-CoA reductase. In 1973 they identified an inhibitor, called compactin, that was made by a relative of the mold from which Alexander Fleming isolated penicillin.
Compactin seemed to have a bright future. Endo joined forces with Goldstein and Brown and other researchers to confirm that it hinders HMG-CoA reductase in human cells. He and other scientists also showed that the compound reduces cholesterol levels in patients with FH. But it would not be the first statin to reach patients. In 1980, Sankyo killed the drug because of worries — which turned out to be unfounded — that it caused cancer. In 1987, Merck’s lovastatin, which was inspired by Endo’s discovery and also derived from a type of fungus, was the first statin approved for treating high LDL.
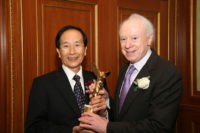
Akira Endo (left) and Joseph Goldstein at the 2008 Lasker Awards ceremony
From their moldy origins, statins have grown into the most important drugs for controlling LDL. They provide dual benefits, reducing the body’s production of cholesterol and spurring cells to install more LDL receptors and extract more LDL from the blood. More than 20 million people now take the drugs, and several studies have demonstrated that they cut the risk of heart attacks and strokes in patients who have high LDL levels. Although compactin never reached the market, a modified version of it lives on as pravastatin, one of the seven statins available in the United States today.
Taking a Bite Out of Hypertension
Like Endo, the 1999 winners of a Lasker Award, David Cushman and Miguel Ondetti, transformed cardiovascular disease treatment by capitalizing on an organism’s chemical talents. The researchers, who were then at the Squibb Institute for Medical Research, devised the first of the drugs known as angiotensin-converting enzyme (ACE) inhibitors, which have been stalwarts of hypertension therapy for 40 years.
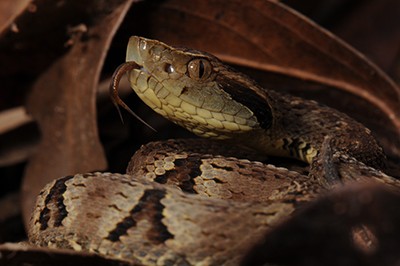
The effect of the venom of Bothrops jararaca inspired Cushman’s and Ondetti’s work.
ACE indirectly boosts blood pressure by switching on a molecule that causes vessels to tighten. The organism that inspired Cushman and Ondetti was a lethal South American snake, the jararaca; its venom causes the opposite effect, a massive decrease in blood pressure.
In the 1960s, scientists began analyzing the venom for molecules that might be useful for fighting hypertension. Cushman and Ondetti also joined the search, and they had an advantage over most other competitors because Cushman had created one of the few assays that could directly determine whether a molecule could inhibit ACE.
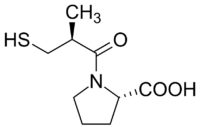
Diagram of the first synthetic ACE inhibitor
In the early 1970s, the researchers deployed their assay to identify a protein shard, known as a peptide, that blocks ACE. The peptide performed well in clinical trials, but it suffered from a serious drawback for a hypertension medicine: it had to be injected. As a result, Squibb decided to abandon it. At that point, “our limited program might easily have died a quiet death,” Cushman recalled in a 1991 retrospective.
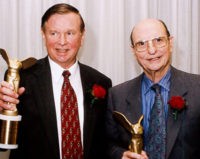
David Cushman (left) and Miguel Ondetti (1999)
“We had not a clue” about how to develop a drug that could be taken as a pill. Cushman’s and Ondetti found that clue in 1974, however, when they read a paper describing a molecule that blocked an enzyme similar to ACE. They saw a new strategy for designing a peptide that could obstruct ACE’s active site and that would be small enough to be taken orally.
Within 18 months they had synthesized captopril, the first ACE inhibitor, which went on the market in the United States in 1981. Captopril was not the first antihypertensive drug, but it was more potent and safer than some of its predecessors. Ten other ACE inhibitors have since been approved, and this class of drugs remains part of “the backbone of hypertension treatment,” notes cardiologist Felix Mahfoud of Saarland University Hospital in Germany.
A Valve Job for the Heart
Alain Carpentier and Albert Starr earned a 2007 Lasker Award for tackling a different aspect of heart disease: the deterioration of heart valves triggered by infections and other causes. Starr was a heart surgeon at what is now the Oregon Health & Science University when, in 1958, a retired engineer, Lowell Edwards, pitched a plan for building an artificial heart. Starr knew that goal was not feasible, but he thought that an artificial heart valve was within reach. The pair decided to focus on crafting a replacement for the mitral valve, which separates the left atrium from the left ventricle and prevents backflow within the heart. Damage to the valve leaves patients exhausted and breathless.
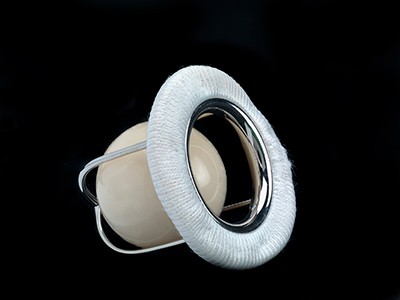
Starr-Edwards heart valve
Image Courtesy of Richard W. Strauss, National Museum of American History
At first Starr and Edwards tried to copy the valve, which has flaps that open and close, but their breakthrough came when they decided to replicate the valve’s function, not its structure. The design they eventually came up with resembles a toy, with a ball inside an elongated cage that has a ring at its base. The ball substitutes for the flaps, floating toward the tip of the cage to allow blood to stream from the atrium into the ventricle and then popping back to seal the opening between the chambers, preventing blood from reversing course.
By 1960, Starr and Edwards had a model ready for patients. They were not the first to build a mechanical heart valve or use the caged ball design, and their creation was not the first implanted into a person. But they were the first to overcome most of the engineering challenges and produce a valve that allowed long-term survival. Although the initial recipient died after 10 hours, the second patient lived for 15 years, and several recipients have now survived for more than 40 years.
Despite these impressive outcomes, Starr and Edwards could not eliminate blood clotting. Patients had to take blood-thinning drugs for life, and even then, some of them suffered paralysis or died from clots spawned by the non-biological materials in the valves. These side effects troubled Alain Carpentier, a resident at Hôpital Broussais in Paris, who decided to take a more natural approach and transplant valves from human hearts. Cadavers proved an unsuitable source, however, so he switched to valves from pigs. In 1965, Carpentier and a colleague inserted the first pig mitral valve into a patient.
Although the procedure was a step forward, the animal valves degenerated within a couple of years, partly because of attacks by the immune system. To solve that problem, Carpentier began studying chemistry at the University of Paris so that he could discover a better way to shield heart valves. He homed in on chemicals called aldehydes, he told an interviewer in 2000, because some of them were used to protect the leather in shoes. Carpentier eventually found that one of the chemicals, glutaraldehyde, made the valves less provocative to the immune system and increased their longevity. In 1968 the first patient received a pig valve treated with glutaraldehyde.
The work by Starr, Carpentier, and Edwards (who was not eligible for a Lasker Award in 2007 because he died before the Award was bestowed) set the stage for the widespread use of prosthetic valves. Almost all of the more than 50,000 valve replacement procedures now performed in the United States every year involve mechanical devices or so-called bioprosthetics derived from animal tissue.
DIY Artery Grafts
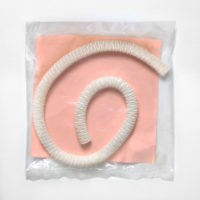
DeBakey-knitted arterial graft of Dacron (synthetic polyester fiber)
Image courtesy of Science History Institute
Cardiovascular surgeon Michael DeBakey of Baylor College of Medicine won a 1963 Lasker Award for developing replacements for another part of the circulatory system, weakened sections of arteries. DeBakey was one of the pioneers of cardiovascular surgery, and in the early 1950s he was trying to devise a method for repairing aneurysms, bulges in the artery wall that can burst and cause fatal bleeding. He started by replacing the faulty artery segments with vessel grafts from cadavers, and in 1952 he and his team became the first in the United States to use this approach to fix an abdominal aneurysm in the aorta, the body’s largest artery.
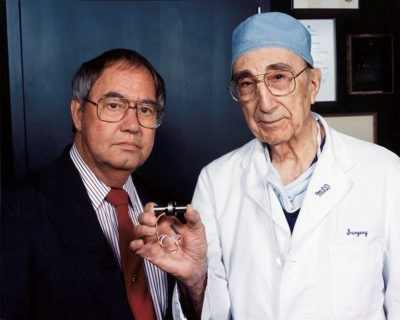
Michael DeBakey (right) holds the MicroMed-DeBakey VAD (ventricular assist device), a tiny heart-assist pump. With him is one of his heart transplant patients, David Saucier, a NASA Johnson Space Center engineer. They helped develop the idea of using Shuttle fuel pump technology to design the device.
Image courtesy of Creative Commons
DeBakey got lucky when he decided to switch to grafts made out of nylon. He visited a local department store, but it was fresh out of nylon. Instead, he walked out with a newer material, Dacron, that proved to be superior for grafts. DeBakey stitched the original Dacron grafts himself on his wife’s sewing machine and implanted the first one into a patient in 1953. Patients are still receiving Dacron grafts today. DeBakey went on to achieve more surgical firsts and to the Lasker Research Awards jury for 30 years.
New Ways to Spare the Heart and Blood Vessels
These four Lasker-winning advances, along with other improvements in treatment and prevention, have made a huge impact on cardiovascular health in recent decades. The death rate from heart disease has fallen almost 70% in the United States since 1950. New approaches may save even more people. “This is a very exciting time” in the field, says Cannon. Here are just a few examples of treatments that have recently reached patients or that are under development.
Drugs that work through different mechanisms than statins can diminish LDL levels even further and help patients who cannot tolerate statins’ side effects. For example, statins target the enzyme HMG-CoA reductase, but researchers found that disrupting another enzyme necessary for making cholesterol, ATP citrate lyase, is also beneficial. The first drug that stifles the enzyme, bempedoic acid, received approval in 2020. Today’s options for patients also include a class of drugs, PCSK9 inhibitors, that block an enzyme that promotes breakdown of LDL receptors. Scientists are now testing a molecule that thwarts PCSK9 and works for six months before patients need another dose.
Because about half of heart attack and stroke victims have normal LDL levels, researchers have also taken aim at other cardiovascular culprits. One example is lipoprotein(a), which also shuttles cholesterol through the blood and promotes cardiovascular disease. A clinical trial is now scrutinizing the first drug that decreases lipoprotein(a) levels. But the target that may have provoked the most excitement is inflammation. Pharmaceutical companies are now scrambling to develop compounds that disrupt molecular pathways that stimulate inflammation, says cardiologist Paul Ridker, also of Harvard Medical School. “That’s a sea change from where the situation was just five years ago.”
One person who was saved by novel therapies, Brown and Goldstein recently discovered, was the girl who sparked their interest in FH. The two researchers lost track of her after their time at the NIH and assumed that, like many people with severe FH, she had died of a heart attack. But Brown says that when he gave a seminar four or five years ago, the girl’s doctor came up afterward to say she was alive.
A community college librarian in Houston, she had taken statins and undergone numerous procedures over the years, including repeated rounds of a dialysis-like therapy to filter excess cholesterol from her blood, as well as a heart bypass operation to replumb clogged arteries feeding her heart. The two scientists finally met her again in 2019, says Goldstein. “She’s an amazing person who’s survived all this time because of advances in cardiovascular disease treatments.”
By Mitchell Leslie