When Richard Nixon declared war on cancer in 1971, the tools to clone human genes were not yet available, so genetic studies of human cancer cells were time-consuming and expensive. The prevailing thought was that bacteria were suitable models for human cells.
“There was a lot of resistance to the idea of beginning to probe the molecular biology of complex human cells,” says Phillip Sharp, who was a postdoctoral researcher at Caltech at the time.
“No one expected to really find anything fundamentally new.”
But Sharp was not convinced. He was intrigued by the observation that RNA inside a cell’s nucleus is much longer than the same RNA outside the nucleus. During his postdoctoral research in 1971, “it seemed clear to me that this long nuclear RNA was a very important phenomenon to understand — either to prove it was merely an artefact and not interesting, or to establish what it was doing,” Sharp says.
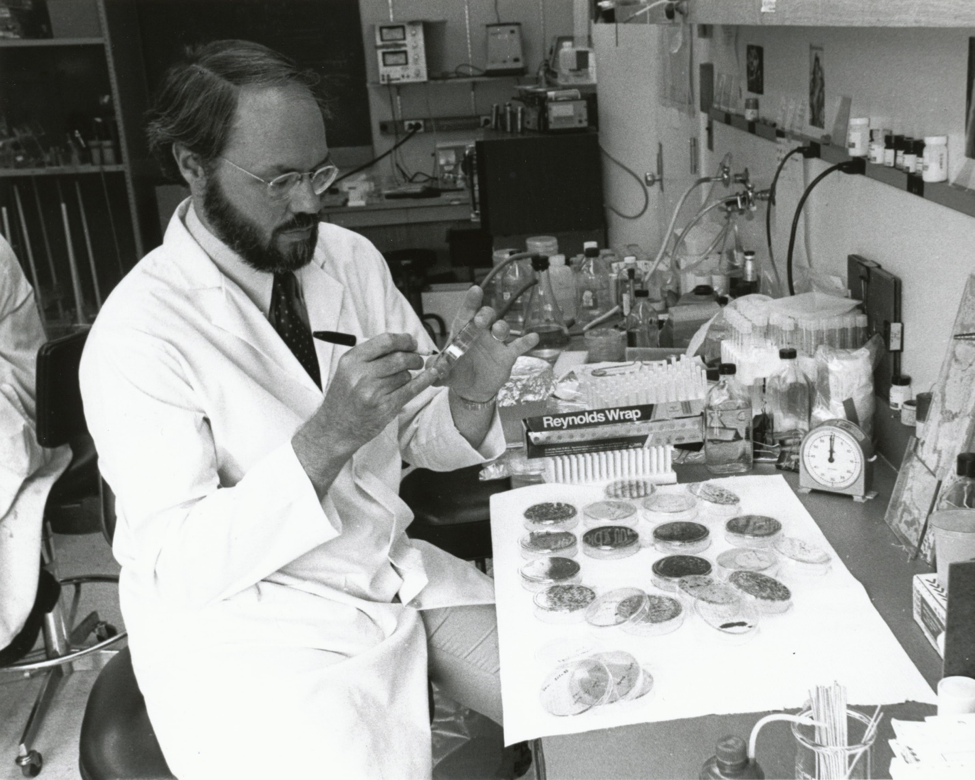
Sharp at work in his lab at MIT in the early 1980s
When he set up his own lab at MIT in 1974, he chose to focus on this mystery of nuclear RNA’s extra length. Within a few short years, his discoveries reworked the foundations of cell biology. They paved the way to understanding the reasons for myriad human diseases, including certain cancers, spinal muscular atrophy, and others. Since 2016, several drugs that target a cell’s RNA machinery have been approved by the US Food and Drug Administration to treat patients with some of these conditions.
Going Viral
As a graduate student in physical chemistry at the University of Illinois, Sharp was fascinated by the polymer characteristics of DNA. But his deep dives into the molecular biology literature revealed that many of DNA’s physical properties were mysteries even in living cells — and their solutions were fundamental to understanding biology. That lured him into molecular biology for his postdoctoral research at Caltech and Cold Spring Harbor, where he began to study how genes mapped onto chromosomes in bacteria and viruses.
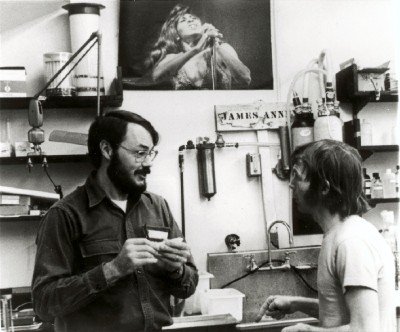
Phillip Sharp (left) in the lab at Cold Spring Harbor with Joseph Sambrook (right)
Sharp collaborated with geneticist Ulf Pettersson, his officemate at Cold Spring Harbor, to study how genes are arranged in the DNA of adenoviruses, a family of viruses that can cause cold-like symptoms or pink eye. They also replicate well in cultured cells. “It seemed an ideal system to investigate this issue between long nuclear RNA and small cytoplasmic RNA,” Sharp says.
Sharp and his postdoctoral researchers chose to focus on an mRNA that encodes a protein in the outer shell of adenoviruses. When they compared the mRNA to its corresponding DNA in the cell nucleus, they found that only parts of the two matched up. In fact, forming a mature mRNA required four separate segments of RNA to be spliced together.
Although their data differed from the conventional thinking that DNA was transcribed directly into RNA, which in turn was translated into a protein, Sharp and his group faced surprisingly little skepticism from their colleagues. “I was surprised that they weren’t more surprised!” Sharp recalls. It turned out that by the time the team presented their data formally, “everyone had data in their notebooks that were explained by this splicing gene structure.”
Sharp’s lab published the first paper to use the term ‘RNA splicing’ in 1977. “And at that stage, the world exploded,” he says.
“It was this breathtaking new phenomenon that was going to rewrite our understanding of genes and gene regulation. It indicated a much more complex system than we had ever understood to exist.”
“It was all extraordinary to me as a new associate professor,” Sharp recalls. “It was overwhelming and terribly exciting. And from there on, it only got more intense.”
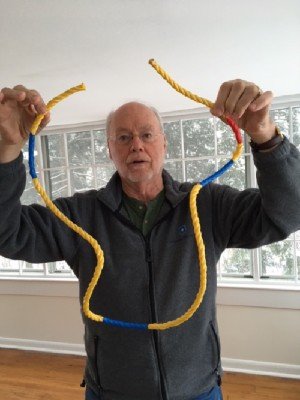
Sharp explains the concept of RNA splicing with rope colored to represent introns and exons.
Rewriting Textbook Science
The discovery of splicing sparked several lines of inquiry as researchers raced to understand how splicing occurs and how it parses genetic information. But Sharp stayed focused on the basic chemistry of RNA for the next 20 years.
With collaborator James Manley, Sharp and his team developed a method for removing introns — the segments of the DNA sequence that aren’t retained in the final RNA — so that they could splice RNA in test tubes for experimental studies. The method allowed the researchers to study the factors necessary for initiating transcription and opened up a new avenue for understanding the mechanistic details of splicing.
In a series of studies, Sharp and his colleagues characterized the structure of introns, showed that a small nuclear RNA component was necessary for splicing, and characterized the molecular machinery required for splicing. Collectively, this body of work “laid down a fundamental understanding of how genes and nuclear processes occur in mammalian cells,” Sharp says. “It’s all in undergraduate textbooks now.”
Beyond the Books
Sharp focused on basic science, but his work has had an unquestionable impact in the clinic. In 1978 — just a year after the first paper on splicing was published — Sharp and others founded Biogen, a Cambridge, Massachusetts–based biotech company. He remained on the company’s board for 29 years and helped lead work that translated the science of RNA splicing into therapeutics.
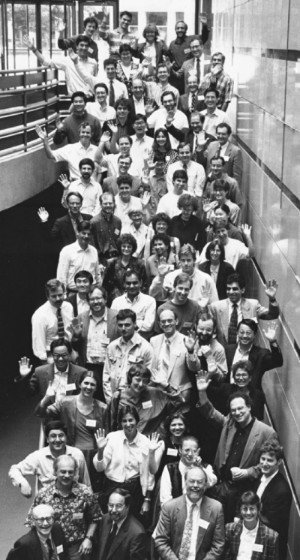
Lasting legacy: A Sharp lab reunion featuring eminent scientists from a wide range of disciplines
Approximately a quarter of the mutations that inactivate human genes impact splicing, Sharp explains.
“All that basic science on the process of splicing is integral to approaches to treating many of our most important genetic diseases,” he adds. “It took us a long time to get there, but now there are at least two approaches to regulate splicing and affect disease outcomes.”
Drugs such as Biogen’s Spinraza (nusinersen) for spinal muscular atrophy (SMA) are antisense oligonucleotides. These compounds mirror the natural RNA found in cells and can bind to it with high affinity. Some of these drugs act by binding to mRNA to inactivate it, whereas others, such as nusinersen, target a splicing site to tweak the mRNA. Patients with SMA have abnormal motor neuron function and suffer developmental delays. Clinical studies of Spinraza show that when administered early, this drug can restore motor function and help slow symptom progression.
Sharp himself remains firmly grounded in basic science and celebrates the benefits it can bring. “Intellectual breakthroughs almost always have implications for therapeutics,” he says. “In fact, the more fundamental the science, the broader its translational impact.”
By Jyoti Madhusoodanan