Susumu Tonegawa is professor of biology and neuroscience at the Picower Institute for Learning and Memory at the Massachusetts Institute of Technology (MIT) and director of the RIKEN Brain Science Institute (BSI) in Saitama, Japan. Tonegawa’s work focuses on unraveling the molecular, cellular, and neural circuit mechanisms that underlie learning and memory. Prior to devoting his career to neuroscience, Tonegawa made important contributions to the field of immunology. In 1987, he received the Albert Lasker Basic Medical Research Award for proving that antibody diversity is the result of an ordered system of gene rearrangement, and for demonstrating that the DNA responsible for antibody production is routinely reorganized to create new genes during the lifetime of an individual. The same year, Tonegawa also won the Nobel Prize for Physiology or Medicine.
Q: After achieving widely recognized success in immunology, how did you decide to switch to working on memory and learning, and how did you make that transition?
A: After having solved what one of the Nobel committee members called the “100-year-old mystery of immunology,” I thought I’d like to continue studying equally mysterious scientific problems that remained unsolved. I chose memory, just one of the mind’s phenomena, as the target of my research because memory is mysterious — memory seems to be at the center of a variety of other mind phenomena like decision, thought, emotion, consciousness. Memory can also be studied with animal models, so it is a viable research target.
In order to understand the neurobiological basis of memory, we must treat the brain as a system and view the behavior of live experimental animals as the output of this system. To study this system in more detail, transgenic and gene knockout technologies seemed to be very promising, as they essentially allow us to (de)activate or label specific brain regions. Fortunately, I had been using these genetic technologies to study some fundamental immunological problems as well, such as immune tolerance. Being able to carry over some of the same research techniques was certainly a great help in my early days of brain science.
Q: The Picower Institute for Learning and Memory has a strong collaboration with the RIKEN BSI in Japan. Can you tell us more about the BSI and in what way is it different from the rest of the Japanese universities?
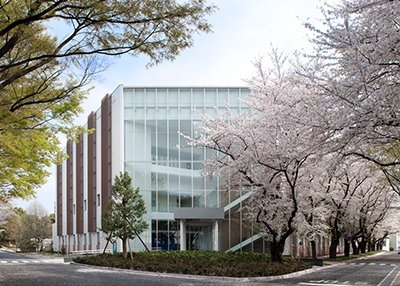
RIKEN Brain Science Institute
A: The RIKEN BSI is a national research institute, and it has its own internal funding. We have made big changes in terms of structure, faculty promotion, and fund distribution since I became the director over 8 years ago. For instance, at BSI, junior faculty members are given complete independence — no hierarchy whatsoever. Also internal funding for junior faculty, in most of the cases, is no less than the funding for senior faculty. So the junior faculty has been very productive, with publications in high-profile journals. In terms of publications, the BSI is as good as the top 10 research centers in the world in brain research, and it’s the best in Japan.
Japanese universities still have quite a bit of hierarchy — there is the big professor, and then the associate professor, and then assistant professors, and so on. They are all hierarchically connected. So young principal investigators (PIs), those scientists who are in their 30s, up to mid-40s, often cannot do completely independent research in many places. This is causing a tremendous waste of talent in Japan. Also younger scientists have to rely on competitive research grants, but the amount they can get is significantly less than what some of the top professors at the top universities can get.
Q: And how do US universities compare in terms of research opportunity, funding, and culture today?
A: One of the strongest reasons why American universities are so productive is because we give freedom to assistant professors, and in a place like MIT, we even try to channel any kind of extra money available at the department or research center to the junior faculty. In the US system, even if obtaining an NIH grant is very hard and even if being an assistant professor is extremely demanding, if the person is talented, they have an opportunity to express their brilliance and talent. When I was very young and I went to graduate school at UCSD, I was really impressed by the horizontal structure among the faculty members and the relationship between faculty members and their students or postdocs. This kind of environment maximizes the expression of scientific talent of younger faculty, postdocs, and students.
Q: Has the research environment changed in Japan since you first left for the United States?
A: It has gotten much better. Since I left for San Diego more than half a century ago, in 1963, the gap between Japan and the US has definitely narrowed. But even today, one of the big problems in Japan is insufficient support for basic research — the government is emphasizing applied research too much. At RIKEN we can still do basic research, but if you go to a national university, of which Japan has about 100, scientists can do first-class basic research studies in only the top 6 or 7 of them. Most of the local universities cannot do good basic research because they don’t have enough funding.
Q: Do you find that there is a similar push here in the United States to fund more applied research?
A: There is always that, but nevertheless, there are sufficient funds for basic research. At MIT, for instance, basic research is ongoing at high volumes. In my lab at MIT, everything we do is basic research. We try to relate the research to the possibility of being useful for some translational research, but when we set up the research proposals, we start with the basic research. History has shown that breakthroughs in therapies or other applications are all based on fundamental research. You can’t have a shortcut in developing new therapies or new technologies. So when I think about the future of Japanese science, I’m worried that the gap will grow during the coming 10–20 years.
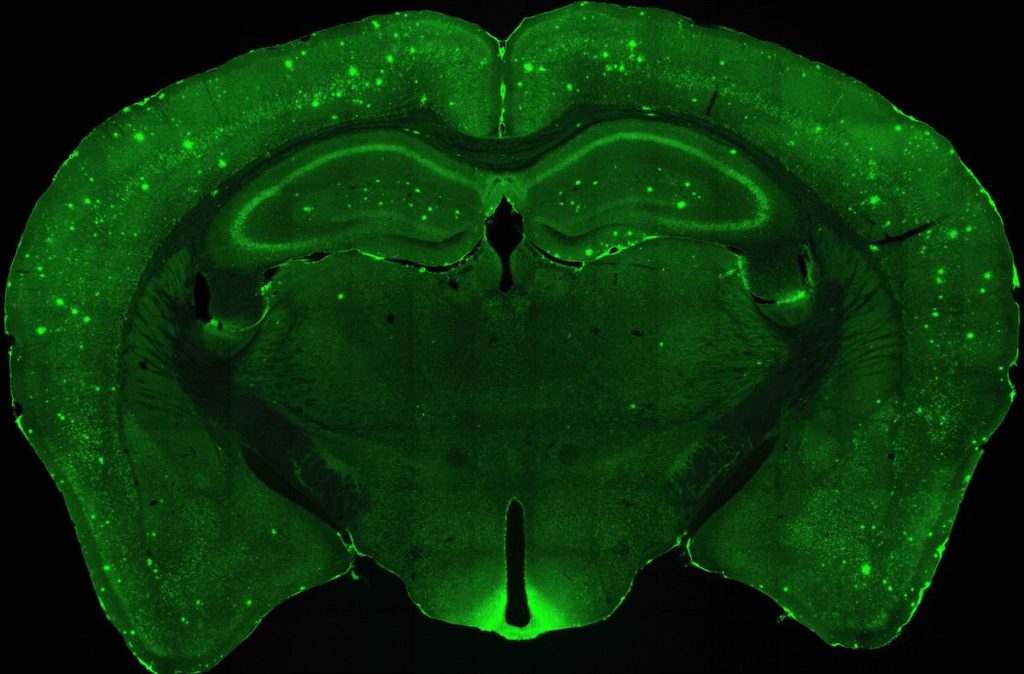
A coronal brain section from a mouse model of early Alzheimer’s disease (AD). These AD mice exhibit severe β-amyloid plaques (green) in the cortex and hippocampus. Credit: Dheeraj Roy. Copyright: d_roy@mit.edu, tonegawa@mit.edu
Q: Under your directorship, the Picower Institute expanded to more than a dozen faculty members coming from quite different research backgrounds. How do you foster collaboration among people with such diverse research interests?
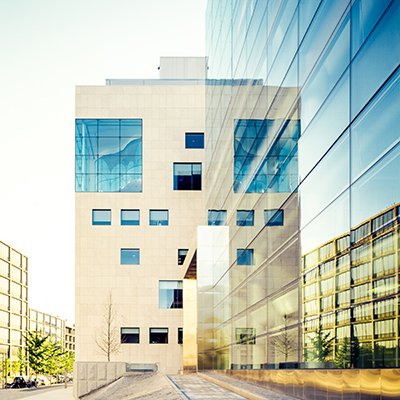
The Picower Institute for Learning and Memory
A: When I started the Center for Learning and Memory at MIT, brain research was becoming so interdisciplinary that we needed to assemble scientists with expertise in a broad range of research. We appointed Matt [Mathew] Wilson, who was an electrical engineer, Earl Miller, who was an experimental psychologist, Morgan Sheng who was a biochemist, and so on. All these people were interested in understanding how memory works, so the intellectual interest was common but their expertise and approaches were complementary.
Q: Can you give us an example of a project that benefited from collaboration?
A: The most recent example is a wonderful collaboration between Picower’s Director Li-Huei Tsai and Ed Boyden [Professor of Biological Engineering] and Emery Brown, who is a computational neuroscientist. They just had a paper in Nature about how to reduce the A-beta plaques in a mouse model of Alzheimer’s. They got together and found a way to reduce the neurodegeneration in Alzheimer’s by shining light of specific wavelengths into the eyes of mice. These lights activate cells in the visual system, which is in the back of the head. Upon entry, the light causes activation of a specific type of cells in the brain, called microglia, which are like immune cells but in the brain. Activation of microglia apparently causes the chewing up of these A-beta proteins. This research could only be done by collaboration with people who have expertise in different fields – from molecular neurobiology, to genetic neurobiology, to engineering, to computational neuroscience.
Q: What are some of the challenges in getting more people to collaborate?
A: I don’t think one should force people to collaborate. You just put the very talented people interested in a common problem under a single roof or in a physically close space and then collaboration often happens. MIT is a very interdisciplinary place. I have a beautiful office here looking over at the Stata Center on the other side of the street. This is where the world’s best electrical engineering computer science department is located. Only one street separates those two top research units, promoting a fusion of biological neuroscience and engineering. In our building we have mathematicians, psychologists, molecular biologists, geneticists, and electrical physiologists, and we are working on research from Drosophila to mouse, to rat, to macaque, to human. I believe such an interdisciplinary environment has a greater chance for highly innovative collaborations.
Q: Over the past decade, or even longer, there has been an expansion in biology research from primarily hypothesis-driven questions to also embrace big data analysis approaches. What is the type of question you ask?
A: As a senior member of the research community, I myself prefer traditional hypothesis-driven research. However, I have nothing against the rise of large data analysis approaches. Hypothesis-driven research is based on the imagination. You think about a problem, you form a hypothesis, and you design an experiment to test whether your hypothesis is right or wrong. This is a wonderful and rewarding exercise, particularly if your hypothesis turns out to be correct. But human imagination is limited. So instead of having your hypothesis dictate your research, you can look at everything in front of you, obtain big data, and use computational power to try to come up with some principle. There are large numbers of people, particularly younger people, who do research on the basis of big data analysis, and I think there is room for both. At least for the years to come, there will be an interaction between those two types of research.
Q: What are the questions that excite you most right now?
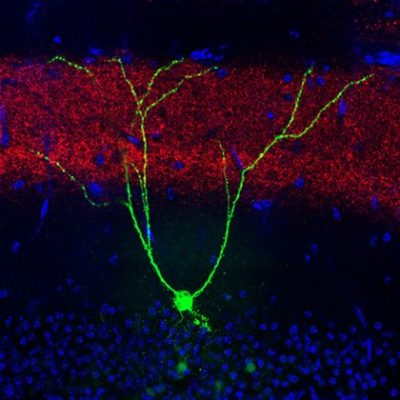
A single memory engram cell (green) in the hippocampal dentate gyrus region of a mouse model of early Alzheimer’s disease. Credit: Dheeraj Roy. Copyright: d_roy@mit.edu, tonegawa@mit.edu
A: My main research interest in the last 15–20 years has been to understand how memory works and how memory fails. I don’t feel this is finished, so I continue to work on various issues related to memory. With more and more precise technologies, we can get a fine map of functional circuits in the brain related to memory function. We have identified what we call memory engram cells – cells that hold specific memory information – and we even have been able to manipulate those cells and alter an animal’s cognition and behavior. We have been able to cure the memory deficits in an Alzheimer’s mouse model or reverse depression in a mouse by manipulating memory engram cells. I hope this basic research will provide the fundamental information that will be useful in the future development of therapies that are not necessarily based on chemical drugs but on direct manipulation of brain cell activity by physical methods such as electrodes, magnetic fields, or even optogenetics.
Another thing is to try to understand cognitive functions such as consciousness and creativity, which have evolved uniquely in humans. We need more noninvasive or low-invasive technology, but since engineering is developing so fast, it is possible that in my own lifetime we may be able to see the glimpse of a beginning of research being done with this type of revolutionary technology that can see inside human brains without any ethical problems. I won’t be surprised if something like this will happen, because there are young brilliant scientists. Students from the electrical engineering department across the street sometimes pop into my office and ask me questions because they are interested in understanding the human brain. That’s the environment we need. In places where biological science and engineering are both excellent, some kind of revolutionary technology will eventually come out. That’s my belief.