May 10, 1979, was a banner day in James Rothman’s lab at Stanford University. A postdoc, Erik Fries, had just finished an experiment yielding the first evidence that Rothman’s unorthodox approach for investigating cells’ protein transportation system worked. Although the evidence was subtle—a wispy line on the readout from the experiment—it was “electrifying,” Rothman later recalled, because most scientists had dismissed his approach as “destined to fail.”
Cells shuttle proteins around inside membrane-cloaked bubbles called vesicles, but in 1979 how cells orchestrate that process, known as protein trafficking, and route vesicles to the right destinations was a mystery. Rothman’s decision to probe protein trafficking in the test tube—rather than in the more complex environment of the cell—allowed him and his team to uncover key parts of the molecular machinery that ensures orderly delivery of proteins within the cell. For those discoveries, Rothman, now at Yale University, earned the 2002 Albert Lasker Basic Medical Research Award and the 2013 Nobel Prize in physiology or medicine.
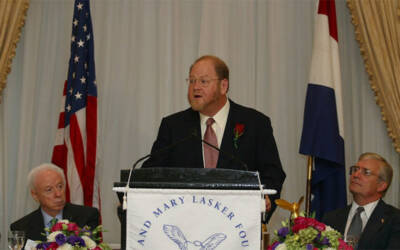
Rothman delivering acceptance remarks at the Lasker Luncheon (2002)
“It took a lot of courage on Rothman’s part to think that he could dissect the biochemistry of something as messy as vesicular transport,” says biochemist Juan Bonifacino of the National Institutes of Health in Bethesda, Maryland. He says that Rothman’s work, along with complementary studies by fellow Lasker and Nobel winner Randy Schekman of the University of California, Berkeley, “set the basis for most later research on the mechanisms of intracellular protein trafficking.” Scientists investigating the transportation system now, including researchers studying how it malfunctions in diseases, “are in effect their children,” Bonifacino adds.
“I Never Wanted to Become a Biochemist”
Had Rothman followed his original ambition, however, his career would have turned out differently. He was born in Haverhill, Massachusetts, in 1950 and wanted to be a scientist “from the earliest time I can remember,” he wrote. By his own admission, as a child he was a science nerd who launched homemade rockets and took over the family pool table to build a centrifuge to test whether g-forces such as those astronauts encounter during their flights affect memory. His pet mouse was his experimental subject. But physics, not the intricate workings of cells, captured his imagination, and when he entered Yale in 1967 he was set on becoming a theoretical physicist.
His plans changed during his junior year when, at the urging of his pediatrician father, he signed up for his first biology class. Rothman was enthralled immediately. Unlike in physics, he recalled, “the research frontier in molecular biology seemed instantly accessible and yet could be equally rigorous and structure-based.”
Rothman has said that his senior year at Yale was when he became a scientist. He took part in a program that allowed him to perform research instead of attending classes. One scientist he worked with that year was Donald Engelman, a biophysicist who had just started a lab at the university. The two investigated the interactions between cholesterol and other lipids in the membrane enclosing the cell. The pair also became friends then and remain close. “What makes him unusual is not just his agility of mind,” Engelman says. “It is that he always focuses on the underlying question of why he is doing whatever he is doing, the deeper meaning of experiments and ideas.”
After graduating from Yale, Rothman again followed his father’s advice and started medical school at Harvard University. He didn’t intend to become a physician. Instead, he saw medical school as an opportunity to fortify his biological knowledge and as a springboard to research. Because he wanted to delve into how neurons communicate, he applied to the neurobiology program. But that program rejected him, and he had to settle for the biochemistry program.
Biochemistry may have been his second choice, but he took to the subject, finishing a PhD project on how cells produce their outer membrane. That research experience proved crucial for his development as a scientist, Rothman wrote. Medical school was “where I became an experimentalist and in particular a professional biochemist.”
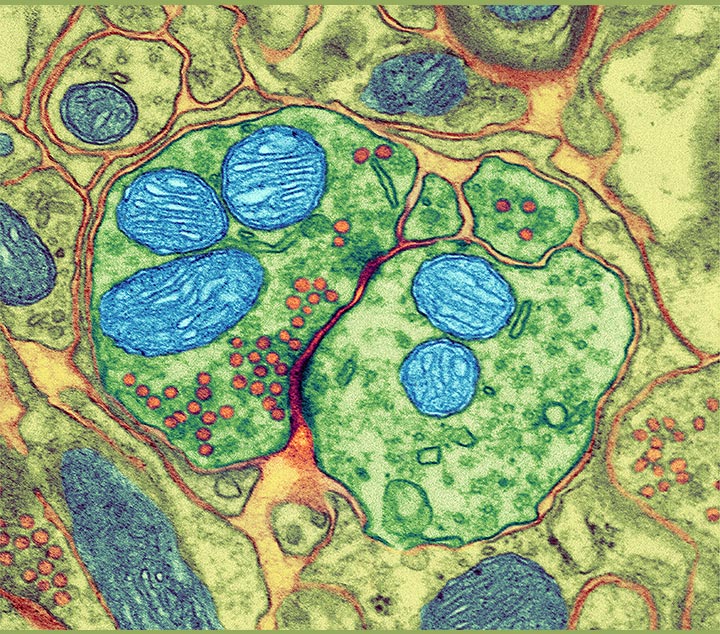
Colored transmission electron micrograph (TEM) of a synapse; the nerve cells are green, with the pre-synaptic cell on the left and the post-synaptic cell on the right. Mitochondria are blue and vesicles are red.
Courtesy of Thomas Deerinck, NCMIR / Science Source
Medical school proved to be a watershed in another way. In a histology lecture he attended his first year, he chanced on the subject that became the focus of his career.
“I fell in love with vesicle transport,”
he said later. For managing logistics, FedEx has nothing on the average cell, which teems with vesicles that ferry an assortment of protein cargoes. A beta cell in the pancreas, for instance, packs newly made insulin molecules into vesicles and dispatches them to the cell membrane. Once there, the vesicles merge with the membrane, releasing the hormone into the cell’s surroundings where it can do its job. Vesicles also ferry proteins between a cell’s organelles. Cells need to carefully control vesicle logistics, and the process falters in many diseases, including type 2 diabetes, Parkinson’s disease, and cystic fibrosis.
In the mid-1970s, however, the mechanics of protein trafficking were mysterious. Researchers didn’t know how vesicles form, travel to the correct locations, or fuse with membranes at their destination. The scientists Rothman described as “the popes” of the field thought that the internal architecture of cells was the key. Certain organelles were close together so that vesicles could travel between them. But when Rothman sketched out how he thought protein trafficking operates, he envisioned a mechanism that didn’t depend on the proximity of specific organelles. Imagining a simple solution for a problem—an ability Rothman attributes to his physics training—has been essential for his career. “Jim is unique among scientists I know in his ability to visualize how things might work,” says structural cell biologist Frederick Hughson of Princeton University in New Jersey.
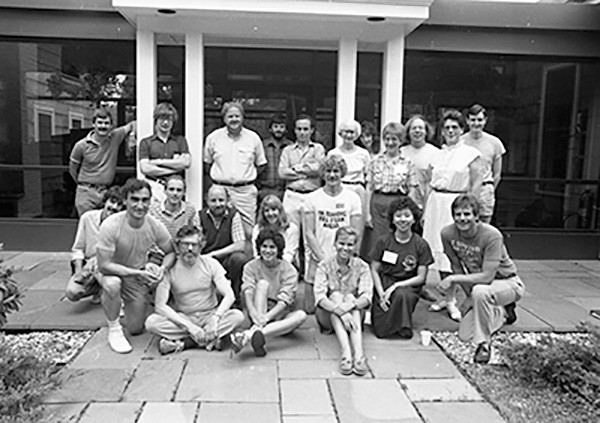
Group photo of Yeast Genetics Course, Rothman is third from left in the standing row (1985).
Courtesy of Cold Spring Harbor Laboratory Archives, NY
No Cells Needed
By the time Rothman started his own lab at Stanford in 1978, he was ready to tackle protein trafficking—and he proposed to study it in the test tube by using the isolated contents of cells. That strategy “was very controversial,” says his former postdoc Suzanne Pfeffer, a molecular and cell biologist at Stanford. Rothman wasn’t the first scientist to use those cell-free extracts, as they’re called, to delve into cellular processes, but other researchers warned that the test tube approach lacked the anatomical arrangements necessary for protein trafficking. But Rothman wasn’t deterred.
“He is a force of nature,”
Pfeffer says. Rothman and his team devised a procedure to test whether vesicle transport occurred in their cell-free extracts. The researchers followed a protein that initially carries sugars that a particular enzyme can cut. In a cell, the protein travels to an organelle, the Golgi apparatus, that progressively modifies those sugars. By the time the protein has finished its journey through the compartments of the Golgi apparatus, those sugars have become impervious to the enzyme. To show trafficking in their cell-free extracts, therefore, all the researchers had to do was detect the enzyme-resistant version of the protein.
In May 1979, Rothman and his team analyzed proteins from their extracts by using a technique known as gel electrophoresis, in which different kinds of proteins separate as they diffuse through a layer of gel, yielding a barcode-like pattern. Scrutinizing that pattern, the researchers noticed a faint line that indicated the enzyme-resistant protein, confirming that vesicles had toted cargoes in the cell-free system. The team presented their findings in several papers in 1980.
Once Rothman and colleagues knew that their extracts reprised vesicle transport, the team could start teasing out details of how the process functions. Pfeffer, who worked in Rothman’s lab around that time, describes the atmosphere as exciting but intense. “He expected a lot,” she recalls. “Every morning, he would ask everyone what they did the day before and what they would do that day. When he had an idea that he wanted to test, Rothman gathered the team to plan and execute a coordinated attack,” she says.
The first question the lab attacked was how vesicles form. Scientists knew that the containers bud from membranes, such as those surrounding organelles, but didn’t understand the mechanism. Rothman and his team followed the process in the Golgi apparatus. They found that what are known as coat proteins congregate at the future budding site and bend part of the membrane into a baglike structure, which then pinches off to become a vesicle. Once the vesicle goes on its way, the coat proteins detach, allowing the vesicle to dock at its destination.
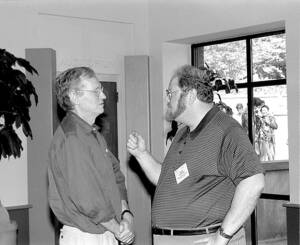
Wolfhard Almers (left) and Rothman at the Cold Spring Harbor Symposium of Quantitative Biology (1995)
Courtesy of Cold Spring Harbor Laboratory Archives, NY
Catching SNAREs
Rothman and his colleagues made some of their most important discoveries while investigating how vesicles find the correct locations to fuse. His group first identified two proteins, NSF and SNAP, necessary for vesicles to merge with membranes. But those proteins couldn’t direct a vesicle to a specific destination. The researchers suspected that membranes must harbor receptors that latch onto SNAP. Using SNAP and NSF as bait, in 1993 the scientists “went fishing,” as Rothman put it, for those receptors in ground-up cow brain. They landed proteins they dubbed SNAREs.
“It’s hard to overstate the impact of the discovery of SNARE proteins,” Hughson says. For example, those proteins explain how vesicles deliver their cargo to the location where it is needed. Vesicles heading for a specific destination carry certain SNARE proteins, and their target membrane boasts matching SNAREs. When a vesicle arrives at the end of its journey, its SNAREs interlock with those on the target membrane, allowing the vesicle to fuse and release its cargo. SNARE proteins also are crucial for transmitting impulses in the nervous system. Nerve cells communicate with each other by releasing chemicals called neurotransmitters, which carry messages across the gap between cells. SNAREs allow neurotransmitter-laden vesicles to fuse with the membrane of the sending cell, thus releasing the neurotransmitters.
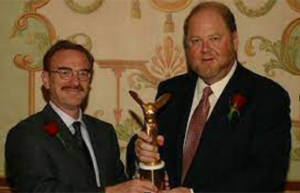
Rothman and Randy Schekman (left) at the Lasker Luncheon (2002)
Working mostly independently from Rothman, Schekman and colleagues discovered that trafficking in yeast operates similarly. The process is comparable in other species as well, researchers have found. The mechanisms that Rothman discovered are significant because they “are general not just to many different organisms but also to many fusion events that take place within the cell,” Bonifacino says.
Today, Rothman continues to probe the intricacies of protein trafficking. Engelman says he’s not surprised that his friend is still hard at work. As Rothman once told an interviewer, he couldn’t imagine not being a scientist.
By Mitchell Leslie