Many transformative biomedical discoveries hit predetermined goals by refining accepted tenets or improving therapeutic practice. But some breakthroughs spring into the hands of alert yet unsuspecting pioneers who wind up shattering conventional wisdom. Lasker Awards have honored several such examples of unanticipated disruptive thinking. For example, Barry Marshall (Lasker Award, 1995) did not plan to upend credos about the origin of ulcers. Thomas Cech (Lasker Award, 1988) did not aim to show that RNA catalyzes chemical reactions, nor did Stanley Prusiner (Lasker Award, 1994) intend to demonstrate that infectious proteins cause deadly neurodegenerative diseases. These revelations materialized when investigators peered at overlooked ideas or dug into provocative correlations. The researchers brought not only creativity, receptivity, and rigor to their projects, but also perseverance and courage.
To succeed, they had to surmount their own skepticism and that of others.
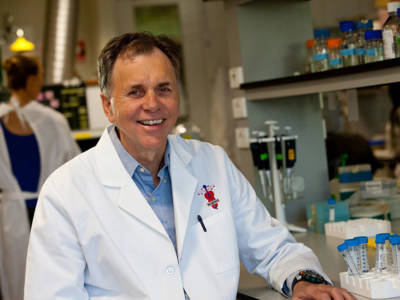
Barry Marshall
Image courtesy of The University of Western Australia
Curiosity rather than purpose can fuel groundbreaking findings. “We didn’t initially chase the cause of ulcers,” nor challenge the decree that they result from stress and diet, says Marshall, now at the University of Western Australia. “We were chasing the possibility that bacteria were living in the stomach.”
In this case, naïveté helped incite work that breached orthodoxy. “I hadn’t been indoctrinated in the principles of gastroenterology,” says Marshall. So in 1981, as an early-career physician, he did not know that medical textbooks declared the stomach too acidic for bacterial survival. When pathologist colleague Robin Warren showed him pictures of spiral bacteria in the stomach, he simply wondered what they were doing there.
The pair found that the microbe, eventually named Helicobacter pylori, appeared in almost all patients who had gastritis or ulcers, and they proposed that it provokes disease. The investigators had not proven causation, however, and colleagues balked, arguing that people with ulcers have “something wrong with their stomach, so why wouldn’t bacteria start living there?” says Marshall.
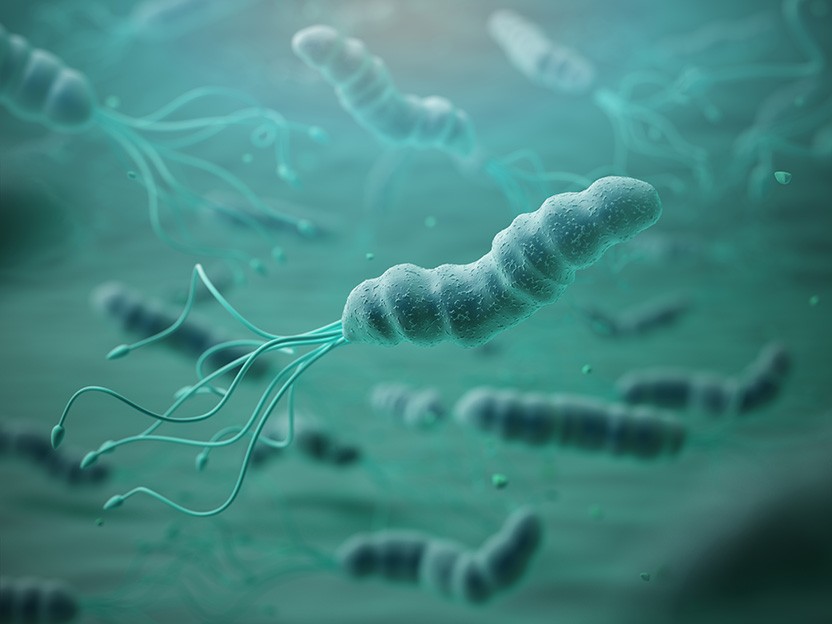
Helicobacter pylori bacteria
Marshall toiled to show that H. pylori triggers inflammation and ulcers in an animal model. After many fruitless attempts, he used himself as a laboratory organism. On June 12, 1984, Marshall gulped down about a billion H. pylori. By the next week, he was vomiting. Tests revealed bacteria in his stomach amidst damaged tissue, which had appeared sound before he drank the microbial brew. H. pylori did not just take advantage of an ailing stomach; it made a healthy person sick. Subsequent clinical trials eventually upheld his idea, and therapies that include antibiotics have transformed ulcers from a chronic, life-threatening disease into a curable condition.
Marshall ascribes the advance not only to his open mind but also to his dual clinician-researcher activities. “Gastroenterologists read the textbooks and look at patients,” he says. “Pathologists see bacteria but don’t know who they come from. Doing bedside to bench gave me a better scientific concept of what was going on inside the patient” — how the presence of bacteria related to symptoms.
Unlike Marshall, Cech and Prusiner initially assumed they were seeking standard solutions to straightforward problems — a protein that removed a chunk of RNA in Cech’s case, a virus that caused a devastating brain disease in Prusiner’s. Ultimately, the scientists’ data forced them to relinquish their initial hypotheses and entertain novel explanations that unsettled entrenched convictions. The ease of convincing others hinged significantly on the degree of confidence their colleagues had in the experimental systems they used.
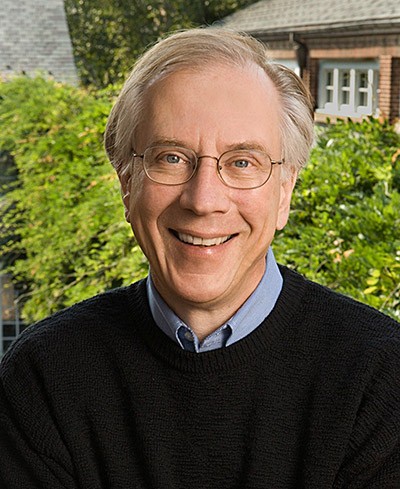
Tom Cech
Image courtesy of Jane Gitschier
In the early 1980s, Cech, at the University of Colorado, Boulder, was studying how a single-celled pond animal called Tetrahymena thermophila makes RNA components of the ribosome, the protein-manufacturing machine. One of these RNAs contained an intervening sequence, or intron, which the cell removes to create the mature molecule. “We wanted to understand the protein enzymes” that performed this splicing process, says Cech.
Proteins were well established as molecules that catalyze chemical reactions, and their 20 amino-acid subunits, each of which performs specific feats, qualifies them especially well for that job. Only proteins, scientists thought, display the repertoire necessary to facilitate the cell’s complex chemistry. Cech requested extra money from the NIH for a cold room, given all of the protein purification he expected to do.
He planned to add material from Tetrahymena nuclei to the precursor RNA that contained the intron. In a stepwise fashion, he would home in on the protein or proteins that performed the reaction. He soon hit a hitch, though. The control sample — with only the RNA and some non-protein reagents — contained as much spliced product as the experimental one. “That was exciting and perplexing at the same time,” Cech says. “It seemed impossible. It seemed extraordinary. It was the opposite of what we expected, but it was absolutely reproducible.”
For a long time, Cech says, “I was wedded to the idea that there was a contaminating protein.” His team exerted tremendous effort trying to detect this theoretical, but—in the end—nonexistent substance. “I don’t take a lot of credit for thinking outside the box,” he says.
“When you’re rattling around inside the box and it isn’t working, eventually you say, ‘let’s change the hypothesis.’”
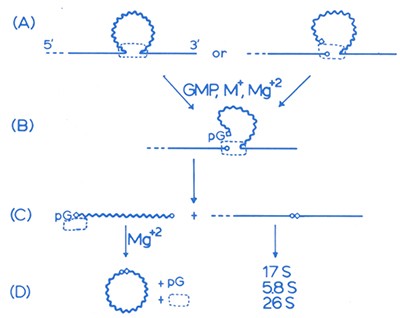
An early drawing of the proposed mechanism of RNA splicing
The dashed rectangle is the hypothetical tightly bound protein catalyst — the one that turned out not to exist.
Image courtesy of Tom Cech
By the time Cech’s group performed the definitive demonstration that RNA could remove its own intron, the result was “almost an anticlimax,” he says. “If you eliminate all other possibilities, what you’re left with — no matter how improbable it may seem — has to be the answer.”
Although experts welcomed his conclusions, some resisted the notion that the observations represented a prototype rather than a quirk. “Now there are many thousands of examples [of the same family of introns] found in many corners of biology — and many other types of RNA catalysis,” says Cech. He attributes the acceptance of his results in part to the fact that the splicing reaction attaches a chemical tag to the RNA, thus providing a clear way to monitor the process, a measurable signal that people trusted. He contrasts this situation to that of Stanley Prusiner with his prions. “A conformation [shape] change is much harder to follow,” Cech says. Indeed, Prusiner and his speculations faced stiff opposition.
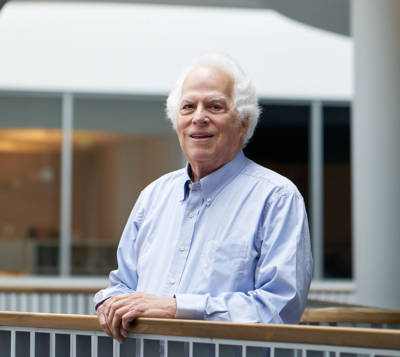
Stanley Prusiner
Image courtesy of UCSF
He, too, followed dogma until mounting evidence forced him to defect. As a neurology resident in the early 1970s, Prusiner, now at the University of California, San Francisco, was puzzled by a patient who died of Creutzfeldt-Jakob disease. The scourge — supposedly due to an ill-defined “slow virus” — destroyed her brain, but without triggering the fever and white-blood-cell surge that usually accompany infectious disease. Soon Prusiner was studying a related sheep malady, scrapie. Others had shown that the pernicious agent endured radiation and other treatments that typically inactivate viruses.
These hints propelled Prusiner on a multidecade odyssey. According to well-tested doctrine, infectious particles need nucleic acid, the genetic material, to replicate. Despite massive efforts, he could not find DNA or RNA in the nefarious substance that apparently multiplied and spread from one cell to another. “I wasn’t a doubter,” says Prusiner. “I never thought I would discover something that went against these kinds of rules.” Protein alone seemed to be the culprit. “Every time I used a technique to destroy a nucleic acid, nothing happened to the infectivity,” he says. “But every time I destroyed protein, the infectivity went away. I kept doing every possible thing I could do to overturn my thoughts.”
He proposed that a protein-based infectious particle — a “prion” — causes scrapie. This model, he wrote in a high-profile paper, “is consistent with the experimental data, but is clearly heretical.” Disbelief flourished.
“People couldn’t fathom” how a protein could act like a virus, Prusiner says.
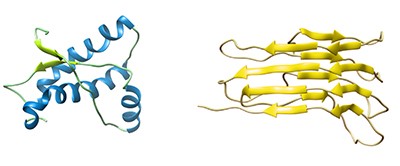
Left: Structure of mouse PrPC (residues 121-230)
Right: A model for the structure of PrPSc (residues 89–280)
Image courtesy of Holger Wille, University of Alberta, Australia
Eventually he showed that a shape change converted the well-behaving version of the protein into a neurological bomb. Still, Prusiner says, he was routinely invited to debate ideas for which he had presented robust evidence. Rather then being treated like a scientist, “I was entertainment.” He almost didn’t get tenure, he says, and at one point, he considered switching careers: “The real estate market was booming.” But researchers who subvert articles of scientific faith often face pushback, he adds. “You have to have the spine to stand up to these people.”
Prusiner traces his fortitude to early nurturing by his mother and grandmother. “Those women gave me unconditional love,” he says. “If you have that, you can withstand anything. If you don’t, things fall apart. I got really lucky.”
Instead of ignoring or abandoning inconvenient observations, these three Lasker Award recipients dug in. Their inquisitiveness, dedication, and independent spirit allowed them to forge new paradigms and conquer resistance within themselves and their professional communities. “If you make an important discovery, you have to be prepared to fight for it,” Marshall says. “People aren’t going to suddenly say, ‘Whoops, we were wrong.’”
By Evelyn Strauss