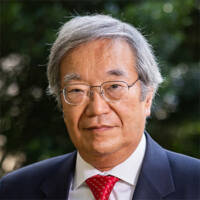
James G. Fujimoto
Massachusetts Institute of Technology
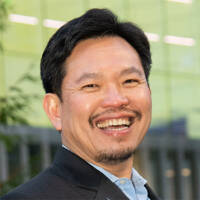
David Huang
Oregon Health & Science University
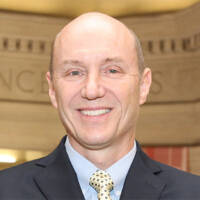
Eric A. Swanson
Massachusetts Institute of Technology
For the invention of optical coherence tomography, a technology that revolutionized ophthalmology—allowing rapid detection of diseases of the retina that impair vision
The 2023 Lasker~DeBakey Clinical Medical Research Award honors James G. Fujimoto (Massachusetts Institute of Technology), David Huang (Casey Eye Institute, Oregon Health & Science University), and Eric A. Swanson (Massachusetts Institute of Technology) for the invention of optical coherence tomography (OCT). This technology uses light beams to visualize microscopic structures within tissues of the body such as the retina. The ability to painlessly generate high-resolution cross-sectional images of the eye’s internal architecture in real time and without physical contact was unprecedented, and OCT revolutionized ophthalmology by allowing doctors to rapidly detect and then treat diseases of the retina that impair vision, thereby saving the eyesight of millions. OCT’s medical use is now expanding, especially because engineers have incorporated it into probes that can enter the circulatory system and they have integrated it into surgical microscopes and other instruments.
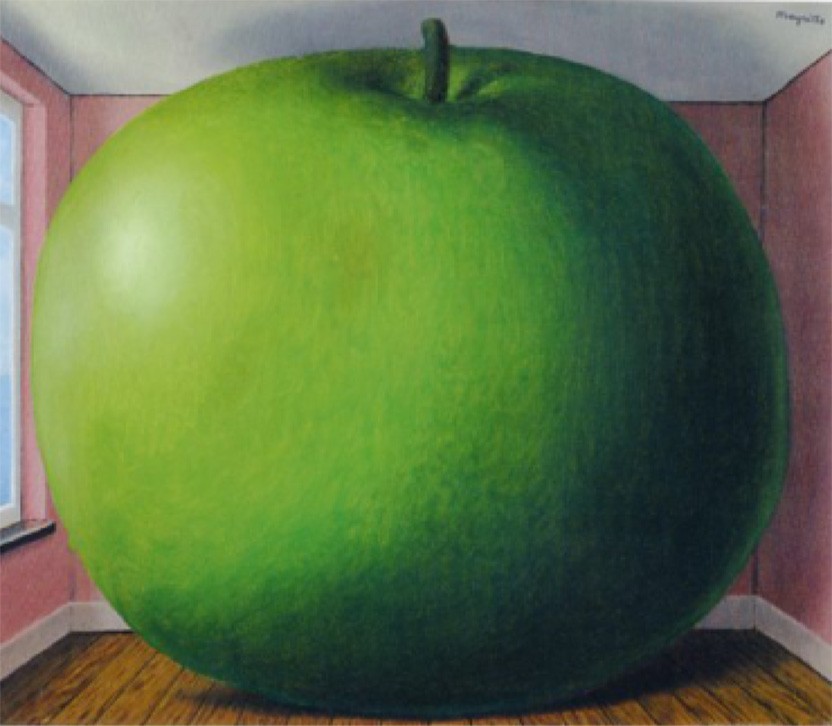
The Secret to a Successful Career in Science—According to Magritte
There is no shortage of words of advice on how to become a successful scientist.
Award Presentation: Michael Brown
The Clinical Lasker Award has long recognized breakthroughs in technology that revolutionized medicine. We honored the inventors of cardiac catheterization, CAT scans, and MRI. This year’s Clinical Award is squarely in that tradition. We honor James Fujimoto, David Huang and Eric Swanson for their invention of ocular coherence tomography, or OCT—a technology that uses light to peer beneath the surface of an object.
In OCT a thin beam of light darts across a surface. The light penetrates beneath the surface and is reflected back to a detector. Objects beneath the surface reflect the light differently so that it returns to the detector with a time delay measured in femtoseconds. A computer converts the time delay into an image that is a cross section of the object. Multiple passes of the light create a series of cross sections. The serial sections can be assembled into a three-dimensional picture. It’s an optical biopsy and it’s better than a surgical biopsy. A surgical biopsy samples only a part of the tissue. OCT shows the whole structure.
Our three honorees built the first OCT machine in 1990 at the Massachusetts Institute of Technology. James Fujimoto is a physicist and optics expert who led the effort. David Huang was an MD/PhD student at Harvard who was pursuing his thesis in Fujimoto’s laboratory. Eric Swanson was an expert in instrumentation working on optics in another laboratory. The three inventors were collaborating with a group of Boston eye doctors. They were trying to visualize the cell layers in the cornea at the front of the eye. It should have been an easy target but they were having problems. So they got the bold idea that their instrument might reveal structures in the retina. The retina is at the back of the eye and was a more complex target. One night they obtained an eye from a calf that was slaughtered. To their amazement, their instrument revealed structures beneath the surface of the bovine retina. OCT was born.
Next, they obtained an eye from a human cadaver. The human retina is only a half millimeter thick. It contains layers of cells including photoreceptors that detect light, pigment cells that nourish the photoreceptors, and neurons that carry visual information to the brain. The retina also contains tiny blood vessels. The OCT machine showed all of these structures. The inventors also obtained a coronary artery from a woman who died of a heart attack. Their OCT machine revealed the deposits of cholesterol and fibrous tissue that had taken this woman’s life. The inventors and their clinical collaborators published their findings in Science in 1991. That paper has been cited 17,000 times.
The initial OCT instrument was slow and not too precise. It was not suitable for clinical use. So the inventors designed a faster and more precise instrument and in 1993 they published the first OCT visualization of the retina of a living human.
To develop their invention, Fujimoto and Swanson helped start a company that they sold to the German optics company Zeiss. Zeiss engineers created precision OCT instruments that can visualize structures as small as one thousandth of a millimeter.
OCT had its major impact on diseases of the retina. From the patient’s standpoint, the procedure is quick and painless. The patient stares into a lens and focuses on a target. The patient sees a thin line of red light that makes many passes across the field of vision. The whole process takes less than a minute. There is no discomfort, and the pupil does not even have to be dilated.
OCT improved the treatment of all retinal diseases including diabetic retinopathy and glaucoma. Its greatest impact has been on age related macular degeneration or AMD which afflicts one in 8 Americans over age 60. The macula is a tiny spot in the middle of the retina where the high acuity photoreceptors are located. This is where we perceive faces, watch television or read newspapers. In AMD the macula degenerates. The photoreceptors are lost and we can no longer recognize our grandchildren. In the wet variety of AMD, the tiny blood vessels in the macula become leaky. Fluid oozes out of the vessel and this causes swelling that distorts the macula. Total blindness occurs.
Fortunately, we have treatments for wet AMD. The 2010 Clinical Lasker Award was presented to Napoleone Ferrara of Genentech for his discovery that macular swelling is caused by a protein called VEGF that causes blood vessels to leak. Ferrara and his colleagues developed Lucentis, a monoclonal antibody that blocks VEGF. When injected into the eye Lucentis stops the fluid leak. The fluid is resorbed, the swelling resolves and vision improves. Another VEGF blocker called Eylea was developed by Regeneron.
VEGF blockers have a problem. Their actions are temporary. After several weeks the fluid recurs and the patient requires another injection. When the VEGF blockers were developed there was no way to tell when the fluid recurred. So the companies recommended that the eye injections be repeated every month. Soon doctors realized that they could use OCT to detect the fluid recurrence, and this would spare unnecessary injections. Currently, patients receive an initial series of 3 monthly injections. After this, they use OCT to examine the eye every month. They give the injections only when the fluid begins to recur. Many patients can go 3 or 4 months between injections. This regimen spares the patient from painful needle sticks in the eye. It also cuts costs. One estimate indicated that by 2015 OCT had saved $9 billion in drug costs.
I will conclude by addressing the most notable aspect of the OCT invention. It illustrates the awesome power of collaboration between physicians, scientists, and engineers. The physicians identified the need. The scientists provided the theory. The engineers built the machine, and the physicians figured out how to use it. The collaboration was energized by a 26 year-old medical student working on a PhD degree. Today clinical/scientific collaboration is threatened. Here are 3 reasons: 1) The public distrusts science. 2) Medical schools are consumed with the economics of patient care. And 3) Companies that invent drugs and devices are vilified as rapacious exploiters of the poor.
Fortunately, we have institutions like the Lasker Foundation that honor science. We also have people like you in this audience who invest their time to learn about discovery. You are our hope for the future. So let’s conclude with a round of applause for our honorees, for the Lasker Foundation, and for you, the enlightened audience.
Acceptance remarks
Acceptance Remarks: James Fujimoto
I come from a middle-class family. We lived in a working-class neighborhood in Chicago. My parents were first-generation Japanese who grew up in California, but because of World War ll, they couldn’t go to college, although they wanted to. That had a big impact on our lives.
My father was an electrician who had a small business. From the time I was five, he’d take me around Chicago to work with him. Because I was small, I helped by squeezing into tight spaces, like ceilings and crawlspaces, where work needed to be done. He taught me a great deal about electrical work as well as how small business operates. But I also got the opportunity to see how other people lived—some struggled, others were incredibly creative, but didn’t have the opportunity to realize their talent.
In my early teens, I built a television from a kit and thought about a career in television repair. However, my life was profoundly changed by a family friend, Tom Tanabe, who played bridge with my father. Tom was one of the few people my parents knew who had gone to college and understood the implications of attending a good university. On bridge nights, Tom would arrive early to talk to me about interesting things, like how ancient Egyptians built pyramids without modern surveying equipment. My father wanted me to attend a local Chicago college but Tom told him MIT was the only engineering school that everyone recognized. He said: “But, of course, you can’t afford to send him there.” My father was so upset that he vowed to send me to MIT if I was accepted.
At that time, I was also studying classical organ at the American Conservatory of Music in Chicago. My teacher, Dr. Amelia Slighting, knew I was torn between a career in music or science. Her advice was that music was very competitive and the possibility of succeeding was very small. She said that although I was talented, she didn’t feel I would reach concert level, and that my artistic interests could also be realized in science. It was hard to leave music behind, knowing that I’d never again reach the level that I had achieved then. But I knew she was right.
I have been fortunate to work with excellent colleagues and students and to have the support of friends and family throughout the years. But it was the influence of my parents, and of Tom, who challenged my father to send me to MIT, and of Dr. Slighting, who advised me to leave music, that are the reasons I am here today. I am forever grateful to have had mentors with great insight into art and science at an early stage in my life.
Acceptance Remarks: David Huang
I was only 26 years old when I started working with Jim Fujimoto and Eric Swanson to develop optical coherence tomography (OCT) and 27 when we published the first paper on this new technology in the journal Science. I was extraordinarily lucky at the start of my career. It was fortunate that Professor Fujimoto assigned me the interferometry project, and that the second tissue we looked at was the retina, which with its many fine internal layers, quickly inspired the idea to develop a novel imaging modality. I was lucky to have worked with Eric, whose photonics wizardry generated prototypes and results in months that would have taken years for a graduate student, if ever!
I was also lucky to run into Joe Izatt, a fellow alumnus of the Fujimoto lab, when I arrived at my first faculty job in Cleveland. Joe helped me rekindle my research career after a 4-year hiatus for clinical training. This was followed by President Clinton’s doubling of the NIH budget in the early 2000s, because of which I was awarded more money than I initially asked for on my first NIH grant, almost a million dollars a year. And Clinton balanced the federal budget! Dr. Michael Chiang, the Director of the National Eye Institute, is in the audience today. I am sure he is thinking too about how we can get back to such great times again.
The OCT field has attracted many of brightest minds because it combines interesting physics, beautiful images, and sight or life-saving applications. I have talked to several leaders in our field who told me that they were working on other physics or engineering projects when they read our OCT paper in Science, and that inspired them to switch direction and focus on this incredibly cool new research subject. In turn I have learned much from their innovations. These conversations have been the most gratifying rewards to me.
Lest you think I lead a charmed life, I want to let you know that I experience the usual share of tribulations. More than half of my grant applications and journal manuscripts have been rejected, and it is not getting better. On the contrary. Just recently, I received a journal review that began: “It’s an honor to review this manuscript … However, in this paper the authors described nothing that would likely to be of any use.” Such reviews arrive regularly whenever we apply OCT to a new tissue target or clinical application. Translational research is tough! It can take years to learn if an innovation is actually useful in the clinic, so my message to others would be to trust your own judgment and not get discouraged by skeptics. It is such a great honor to be recognized with the Lasker~DeBakey Award. Thank you!
Acceptance Remarks: Eric Swanson
Although it was over 30 years ago, I vividly remember the world’s first in vivo human retinal OCT image. After we received IRB approval from MIT to start human studies, I took a shuttle bus from MIT Lincoln Laboratory where I worked to the MIT campus to join Jim and our colleagues to test the equipment. On the ride in I wondered: Who will go first? Is it really safe to shine our laser into someone’s eye and focus it right on their retina? Were the safety calculations really done correctly? Then, in a small room outside Professor Fujimoto’s office we all sat down and without hesitation Jim took off his glasses and put his chin on the chin rest. I thought WOW, that was courageous! And it was safe — and it is safe — and out came a beautiful real-time image!
Part of what made that moment so special for an engineer is that OCT systems involve many different technologies, including optics, photonics, electronics, software, image processing, and mechanical systems. As a team we got to focus, not just on one main technology, but many and to put them together into a system that had an important purpose. It is one of the reasons that the field of OCT is so much fun to work in. I think everyone who has built an OCT system feels this sense of wonderment, excitement, and satisfaction the first time they acquire an image.
When you have the good fortune of collaborating in a field where there is a lot of room for innovation and opportunity for impact, the lines between work time and personal time become blurry. Working late into the night or on weekends on OCT was not a burden, it was enjoyable. I believe it’s an important clue for young people that, when work and discretionary time feel the same, they are on the right career path.
The discovery and advancement of OCT was powered by a complex ecosystem involving: substantial government funding for basic research; the collaborative and competitive nature of research; the creation of multi-disciplinary teams of scientists, engineers, and clinicians; and the power of entrepreneurship and industry. Today there over one hundred companies making OCT technology. Many originated as startups, including two I co-founded, a clear testament to the entrepreneurial spirit and the positive impact entrepreneurism can have in expediting translation from research into clinical care.
Tens of millions of OCT procedures are performed every year, and a healthy ecosystem with thousands of innovative researchers, engineers and business people is advancing the field. OCT is a broad and powerful technology that will continue to have a positive effect on society – so many wonderful things are still to come.