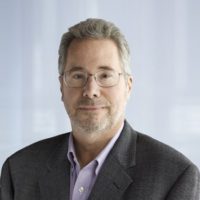
Richard H. Scheller
Genentech
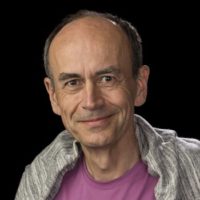
Thomas C. Südhof
Stanford University School of Medicine
The 2013 Albert Lasker Basic Medical Research Award honors two scientists for their discoveries concerning rapid neurotransmitter release, a process that underlies all of the brain’s activities. Richard H. Scheller (Genentech) and Thomas C. Südhof (Stanford University School of Medicine) identified and isolated many of this reaction’s key elements, unraveled central aspects of its fundamental mechanism, and deciphered how cells govern it with extreme precision. These advances have provided a molecular framework for understanding some of the most devastating disorders that afflict humans as well as normal functions such as learning and memory.
The billions of nerve cells in our brains allow us to savor chocolate, whack a baseball, and imagine traveling at the speed of light. Their exploits tell our hearts to quicken and make us feel as if those same hearts are breaking. Their messages give us eureka moments — and let us jump out of the bathtub in response.
Award presentation by Eric Kandel
We are who we are because of our brain and its ability to acquire and store new information. This ability derives from the remarkable capabilities of the 100 billion signaling units in the brain called nerve cells — or neurons — and in particular from the ability of these nerve cells to communicate with one another through specialized contact points called synapses. A synapse has three components: a pre-synaptic terminal contributed by the sending neuron, a post-synaptic receptive component contributed by the receiving cell and a synaptic cleft — the space between the pre- and post-synaptic components. Synapses operate by means of a chemical called a neurotransmitter that is released from the presynaptic terminal by a process involving the influx of Ca2+, whose molecular bases our two Lasker Basic Science Prize winners, Richard Scheller and Thomas Sudhof, have pioneered in defining.
Acceptance remarks
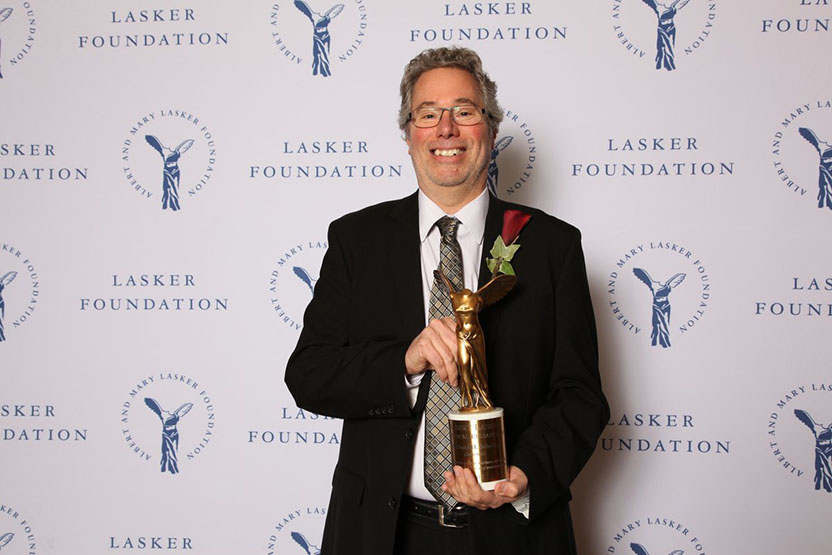
Acceptance remarks, 2013 Lasker Awards Ceremony
I would like to take a couple of minutes to reflect on the progress made in cellular and molecular neuroscience and to then comment on what I see as some of the biggest problems facing the field, particularly in the area of human health.
I went back and looked at the proceedings of the first National Institute of Mental Health Conference on Molecular Neurobiology that was held in Santa Barbara, California, 25 years ago. Steve Zalcman and I organized the conference, and I’m happy to note that Steve is in the audience today. The problems we were attempting to solve were not new but we were armed with a new set of molecular, cellular, and genetic techniques that gave us hope for unprecedented progress. Interestingly, the field was relatively small at the time. Most of the important people in the field fit into a medium sized room, all spoke during the three-day conference, and many are here today.
My first observation is that the breadth of this field has grown immensely such that topics represented 25 years ago by individual speakers would now require individual meetings.
Second, while it may not feel that way day to day in the lab, in my opinion progress has been meteoric. This is confirmed by the fact that 5 of the people who attended that first conference have since won the Nobel Prize and others have received the Lasker, Kavli, Gardner, Gruber, and other prizes. We have gained tremendous insight, or essentially solved, many important problems in the field. I do not have time to go over that progress in detail, which in any case does not need to be recounted for this learned audience.
Third, when I think about how this progress will be applied to the important medical problems facing mankind, I am both excited and a bit concerned.
I believe that we have a number of insights that provide those of us interested in therapeutic intervention reason to be optimistic about our progress working with diseases of neuronal degeneration, such as Alzheimer’s, ALS, and Parkinson’s. The genetics of these conditions has uncovered pathways that are likely important in causing the diseases, at least in certain subsets of patients. The ability to dissect the pathways with molecular and cellular techniques has pointed us toward mechanism and in some cases suggested ways one might intervene with therapeutics.
In contrast, I am much less optimistic about our ability to make rapid medical progress in psychiatric disorders such as depression, schizophrenia, and autism. These diseases manifest themselves through the immense complexity of neural circuitry and are perhaps neurodevelopmental disorders. Whatever their origin, we need a much greater understanding of the brain before we will have a mechanistic understanding of the cause of these psychiatric disorders which will then guide us towards effective treatments.
What do we do about this? I have no answers except a trivial solution. Keep working hard. I believe that if the progress in the next 25 years is as tremendous as the last 25 years we will have insights into these devastating diseases that will provide major benefits for patients and society.
Finally, conducting this research is expensive. Unfortunately, over all NIH funding, corrected for inflation, peaked about a decade ago and has even more dramatically decreased on a per laboratory basis. I know many of us hope this trend will be reversed so the promise of understanding the brain and its devastating diseases can be realized.
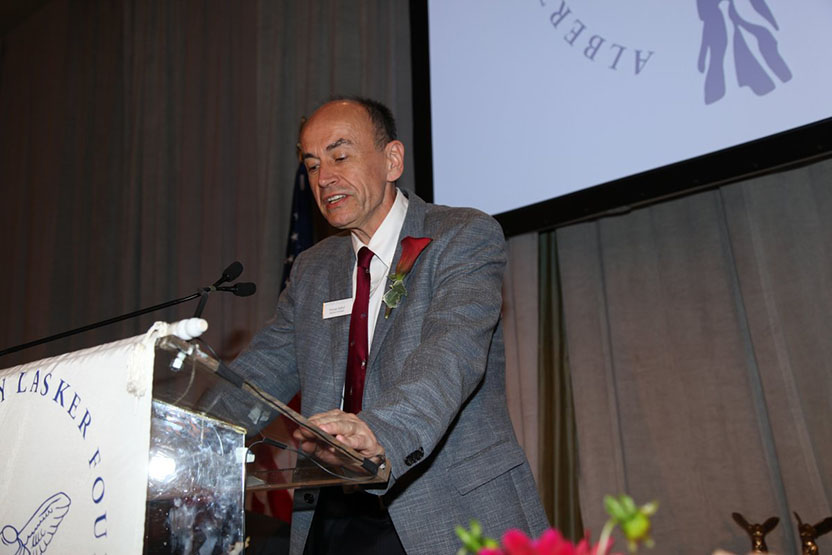
Acceptance remarks, 2013 Lasker Awards Ceremony
I grew up in an academic household with a strong religious bent. True to my name and nature, from early on I doubted the tenets of my parents’ beliefs — I probably was not easy to have around (hasn’t changed …). What my upbringing instilled in me was a desire to question what is actually true. My greatest pleasure has always been to discover facts, to figure out how something works, to identify the relationships and connections that explain an observation. I feel particularly honored by this award because the Lasker Award recognizes long-lived advances of medical knowledge that produce true insights, rather than some short-lived intriguing “discovery” that appears on the front page of the New York Times.
I studied medicine because I wanted to do something useful, but I soon started to do science on the side. Initially, I was a biophysicist and biochemist who studied organelles and proteins in Victor Whittaker’s laboratory in Göttingen. Whittaker was the scientist who first isolated synaptic vesicles in the 1960s. Shortly after finishing medical school in 1982, I became a postdoctoral fellow with Mike Brown and Joe Goldstein at UT Southwestern in Dallas, where I learned to be a molecular cell biologist and studied the LDL receptor gene and cholesterol regulation. After finishing my post-doc, I merged my earlier love of synaptic vesicles with my new knowledge of how to purify and clone membrane proteins, with the ultimate goal of pursuing something entirely new — to identify all the membrane proteins that make up a synaptic vesicle, and to figure out how these vesicle proteins mediate release of neurotransmitters.
Synaptic vesicles are chock-full with neurotransmitters and release them by fusing with the presynaptic plasma membrane. Neurotransmitter release underlies all communication of neurons with each other at synapses. When I began this work in 1986, none of the proteins of synaptic vesicles had been identified. Nothing was known about neurotransmitter release except that it was triggered by calcium. Whittaker had introduced me to the subject of synaptic vesicles and neurotransmitter release, but it was Joe Goldstein and Mike Brown who had taught me how to actually approach this subject, and how to study how a synapse works.
Over the last 25 years, I mutated not only mouse genes, but changed myself, morphing from a molecular biochemist into a mouse geneticist into an electrophysiologist into a behavioral neuroscientist. These changes gave me the pleasure of always learning something new about how synaptic vesicles and synapses work, although these changes unfortunately have not improved my looks.
The 1990ies were an amazingly thrilling time! During this time, we together with our long-time collaborators Reinhard Jahn and Jose Rizo, and in parallel with Richard Scheller and others, discovered the role of SNARE and SM proteins in synaptic vesicle fusion. We identified synaptotagmins as calcium-sensors in neurotransmitter release, showed how synapses are organized by active zone proteins, and described the first synaptic cell-adhesion molecules that guide synapse formation. These discoveries and conceptual advances were the beginning of an understanding of synapses, which has now become generally recognized. During the course of our work, the studies of Jim Rothman, who received a Lasker Award in 2002 for his work on the cell biology of vesicle fusion, expanded our thinking and injected great enthusiasm into the rapidly growing field of neurotransmitter release.
I thank the Lasker Foundation — I thank you all for giving me this award, and I hope to live up to it!
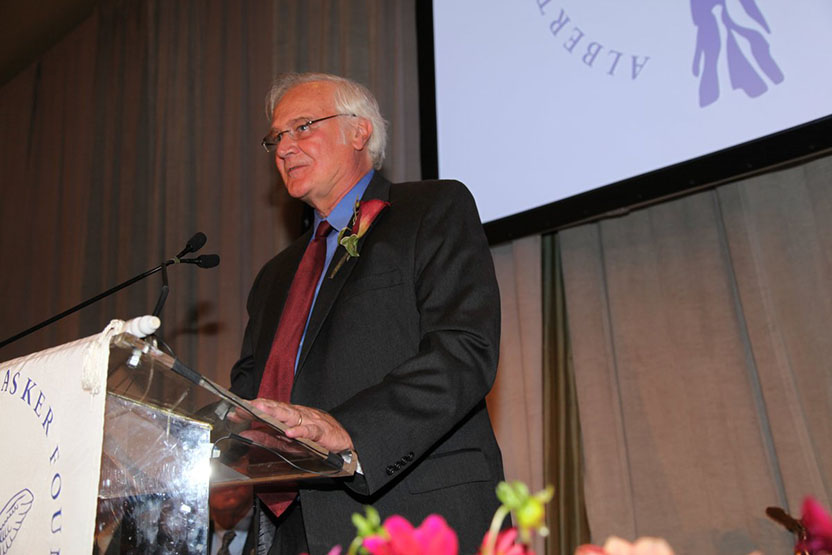
Acceptance remarks, 2013 Lasker Awards Ceremony
Development of the modern cochlear implant was a worldwide effort involving many scientists, engineers, physician-scientists, and research subjects. The success of this effort is an outstanding example of the power of collaborations between the public and private sectors and also the informed support by the NIH of applied as well as basic research.
I am proud to stand before you today as a representative of the worldwide effort, and I am especially proud to stand with Graeme Clark and Ingeborg Hochmair, who are two of my heroes and the foremost living pioneers in our field.
Although the present cochlear implants are truly wonderful, room still exists for improvements. A variability in outcomes remains, and even the top-performing patients experience difficulties in understanding speech in adverse acoustic environments such as noisy restaurants or workplaces. In addition, reception of sounds more complex than speech — such as music — is less than satisfying for most patients. Research is underway to narrow or even eliminate these gaps between prosthetic and fully functional hearing, and to narrow the range of outcomes such that all patients will achieve high levels of performance. Many promising possibilities are being pursued by extraordinarily talented investigators, and I am completely confident that further improvements will be made.
An even more important challenge — in my mind — is to make the highly effective technology we have today available to all persons who could benefit from it. Thus far, about 320 thousand persons have received a cochlear implant in one or both ears. But various estimates indicate that as many as 25 million persons worldwide could benefit from a cochlear implant. That means that only about 1 or 2 percent of the population who could benefit actually have received a cochlear implant.
The cochlear implant is a transformative technology that allows children to be mainstreamed into regular schools, adults to have a wide range of job opportunities, and all recipients to connect in new and important ways with their families, friends, and society at large. The resulting human and economic benefits are immense.
In many parts of the world, cost is a barrier to widespread applications of the technology, even though the benefits ultimately far outweigh the cost. The principal expenses are in providing the appropriate medical infrastructure and care. The cost of the device also plays a role, but that cost is coming down and is not the dominant factor for most countries. Several of us in this room are working to reduce or remove the cost barrier, and to improve hearing health care worldwide, which includes prevention, screening, and treatments in addition to cochlear implants.
This magnificent award will greatly increase awareness of how cochlear implants can enable severely and profoundly deaf persons to realize their full potential in life, and that awareness will in turn facilitate further dissemination and development of this marvelous technology. Thank you for welcoming Graeme, Ingeborg, and me into the Lasker family, and thank you for the highly favorable tailwind you have given us and our colleagues to do more!
Interview with Richard H. Scheller and Thomas C. Südhof
Video Credit: Susan Hadary