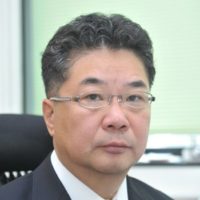
Kazutoshi Mori
Kyoto University
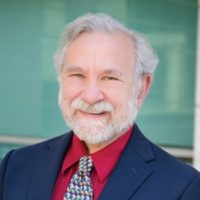
Peter Walter
University of California, San Francisco
The 2014 Albert Lasker Basic Medical Research Award honors two scientists for their discoveries concerning the unfolded protein response, an intracellular quality-control system that detects harmful misfolded proteins in the endoplasmic reticulum and signals the nucleus to carry out corrective measures. Kazutoshi Mori (Kyoto University) and Peter Walter (University of California, San Francisco) identified core components of this process and unveiled unexpected aspects of its mechanism.
Approximately one-third of cellular proteins pass through the endoplasmic reticulum (ER), a netlike labyrinth of membrane-bound tubes and flattened sacs inside the cell. Work in the 1960s revealed that the ER sorts and transports proteins that are destined for export or the cell’s surface, and we now know that the ER allows cargo to pass only after applying stringent standards. In particular, proteins must assume correct three-dimensional shapes to perform their jobs, and the ER fosters this outcome. Furthermore, when unfolded proteins accumulate in this compartment, the cell bolsters the ER’s folding capacity. This phenomenon forms the linchpin of the unfolded protein response (UPR).
Award presentation by Michael Brown
Henry Ford is credited with inventing the assembly line, but Mother Nature beat him by a billion years when she invented the endoplasmic reticulum, Mother Nature’s assembly line. In Ford’s assembly line parts are passed from one worker to another. At the end of the line is the inspector, who tests the product. Correctly assembled products are exported. Defective products are destroyed. If too many products are defective, the inspector sends a signal to the control room, where supervisors dispatch troubleshooters to correct the problem.
Ford never knew he was copying the endoplasmic reticulum, which I will abbreviate as ER. The ER is a network of membrane-bound tunnels within every nucleated cell from yeast to human. Like Ford’s assembly line, the ER makes products for export. Its products are proteins designed to function not in the controlled environment of the cell, but in the harsh environment outside. Some of the proteins are destined to reside on the cell surface, where they serve as receptors that respond to external signals. Other proteins are secreted to influence the behavior of other cells. Lasker laureate Günter Blobel showed that exported proteins are inserted into the ER during their translation. Once inside, they are bound by the factory workers of the ER, which are proteins called chaperones. Like Ford’s welders, the chaperones help the exported proteins to fold into their final three-dimensional structures. The ER assembly line must perform perfectly. Mistakes are not tolerated. Unlike in the auto industry, in biology there are no recalls.
Acceptance remarks
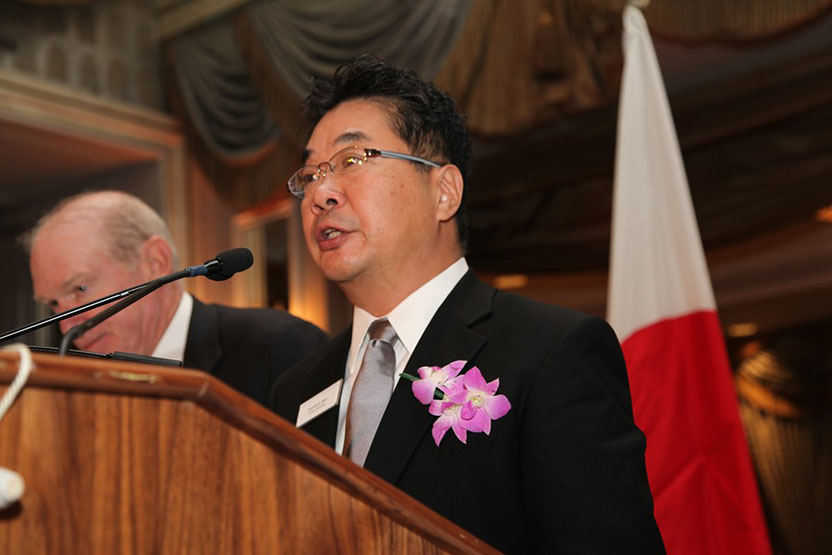
Acceptance remarks, 2014 Lasker Awards Ceremony
It is my very great pleasure and honor to receive the Lasker Award. Looking back, I can point to many happy meetings that changed the directions of my career. In particular, three meetings come to mind. The first happy meeting took me to the United States. I did not like biology in high school. Textbooks seemed descriptive. I felt I had to learn everything by heart. As a freshman of Kyoto University, most of my learning came from newspapers. I was amazed by the fact that the genetic code is conserved from Escherichia coli to humans, which allowed scientists to produce human proteins in E. coli. I hoped for a bright future in biology. So, I switched my major from chemistry to biology.
Since there was no molecular biology program at my school, I studied biochemistry. After graduating, I obtained a permanent position at a local university. This was very fortunate because Japan had no post-doc system at that time. I worked hard but did not enjoy the biochemical project I pursued — I wanted to do something more interesting and important. I decided to quit the position and go to United States, where I had my first happy meeting: in 1989, at the University of Texas Southwestern Medical Center, I met the discipline of molecular biology under the mentorship of Mary-Jane Gething and Joe Sambrook.
There, in Dallas,Texas, I was introduced to the unfolded protein response (UPR) — my second happy meeting. I succeeded in cloning IRE1, which turned out to be a key molecule to unraveling the UPR in yeast. To my surprise, Peter Walter came up with the same molecule at the same time. In our first papers, published back to back in Cell, Peter and I discovered that IRE1 had a strong kinase activity.
After four-and-a-half years in Texas, I came back to Japan and found a position at the HSP Research Institute in Kyoto. My next targets were transcription factors specific to yeast UPR. My new director advised that I be careful: IRE1 is a kinase, he said, and there may be a downstream kinase cascade. So, you might end up obtaining many kinases but no transcription factor. And because the Institute focuses on transcription, we do not want kinases. He told me to think of a method of obtaining a transcription factor directly. But this was a difficult task. After a 14-month struggle, I finally came up with a simple method called one-hybrid screening, which turned out to be the right approach. It worked magnificently, and in 1996, I identified HAC1, the key transcription factor for the UPR In yeast. And with my one-hybrid screening approach, several years later my colleagues and I obtained two other crucial transcription factors for the UPR in human and animal cells.
Had I stayed in the United States after my Texas days, I would have probably employed a complicated American-style screening approach that would have never allowed me to keep up with a major player like Peter Walter. But the development of my simple one-hybrid screening was my third happy meeting — to me, it was the Japanese version of the American dream. Thank you again for honoring me with the Lasker Award.
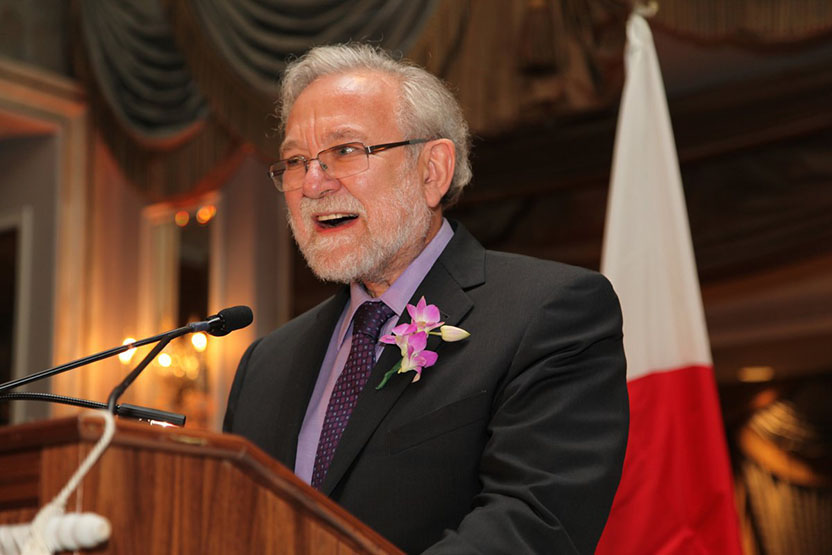
Acceptance remarks, 2014 Lasker Awards Ceremony
Four years ago, Joe Goldstein issued the ultimate instructions on “How to Win a Lasker.” In his short piece in Nature Medicine, he presents a list of advice, “culled from the experts,” as he puts it, and then promptly dismisses it as a “not foolproof formula.” He continues to quote as better advice the British mathematician Godfrey Hardy, who defined in the early 1900s scientific beauty as an art form in which outstanding science gives you “cerebral chills and intellectual kicks” — that combine the qualities of significance, generality, and unexpectedness.
Biology, however, is not mathematics, nor is it physics, nor chemistry. As biologists, we are not free to impose our own axioms to inject beauty into our work or even to assume elegant adherence to deterministic logic. In biology, Mother Nature presents the playing field, and it is our task to decipher how it works. Disconcertingly, Nature deploys the strategy of random walk, of mutation and selection, leading to the evolution of the world that surrounds us. She then presents us with the most fascinating puzzles to decipher: the inherently unpredictable Rube Goldberg machines that make up a living cell.
When we began working on the unfolded protein response that we celebrate here today, we found ourselves traveling along a road that seemed comfortable and predictable, perhaps too narrow and straight. We asked but simple questions: “how does one part of the cell know what is happening in another?” And, we got a simple answer: a kinase receptor that, like many others, controls the cell’s gene expression system. But suddenly, the ground shifted, and we stepped into a morass, in which the seemingly familiar dots that before gave us a sense of security no longer connected. Walking on, undeterred, we deciphered one of most unusual cell-internal communication pathways, made up almost entirely from an unprecedented potpourri of repurposed components. To top it off, the salient features of what we learned from simple, single-celled brewer’s yeast holds true for our own cells, and these features now emerge as impacting players in a plethora of human diseases — giving us hope that our esoteric findings will be translated one day into tangible benefits for mankind.
My main point here is that none of this was predictable. Neither I nor Kazu stand here today as genius mathematicians akin to Hardy or artists, who begin with an empty canvas and control over the elegance of their work. We are explorers — not designers — facing the chaotic randomness of evolution. We diligently deciphered one of Nature’s guarded mysteries, and only then could we condense our findings to their most elementary beauty. To us, it has been a fantastic journey filled with adventure, cerebral chills, and intellectual kicks. Yes, this journey required radical thinking and fearless experimentation. But only in the end, did it all combine — serendipitously — into a story of unexpectedness and, we hope, lasting significance and generality.
We are thrilled to be here today, and we deeply appreciate that our work has been so well received.
Thank you for such a nice celebration.
A toast to discovery!
Interview with Kazutoshi Mori and Peter Walter
Video Credit: Susan Hadary