One day in 1978, Robert Furchgott’s lab technician made what turned out to be a momentous blunder. Furchgott, a pharmacologist at what was then the State University of New York Downtown Medical Center in New York City, and his team had been probing how certain drugs and molecules affect the circulatory system. Their procedure involved applying the chemical in question to a piece of tissue carved from an artery to see whether the snippet contracted or relaxed.
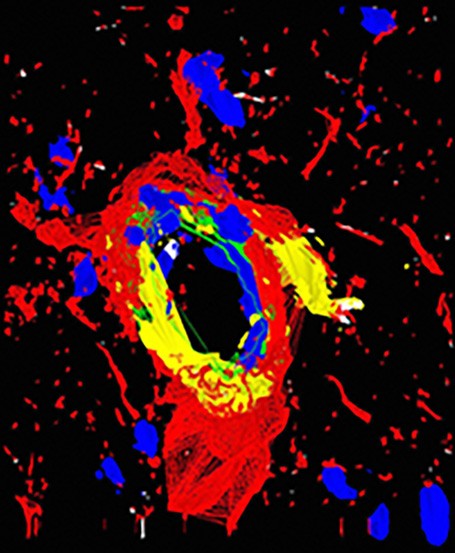
3D fluorescence deconvolution micrograph of a section through a dilated arteriole blood vessel from the olfactory bulb of the brain. Endothelial nitric oxide, a powerful vasodilator, is red. Increasing the diameter of the arteriole increases the blood flow to the olfactory bulb.
Courtesy of Roger J. Bick & Brian J. Poindexter / UT-Houston Medical School / Science Source
On that day, however, Furchgott’s technician prepared the tissue incorrectly. The arterial piece relaxed when exposed to a molecule called acetylcholine. That result was disconcerting because in the 25 years that Furchgott and colleagues had performed similar experiments, acetylcholine had always prompted the arterial slice to contract. Puzzled, Furchgott began investigating the discrepancy, and his research over the next few years led him to propose the audacious hypothesis that nitric oxide (NO) is an important chemical messenger for communication among cells.
NO is a toxic gas, so his proposal didn’t sit well with many scientists.
“Nobody in their right mind would think that nature would use that molecule to be a signaling molecule,”
says biochemist Michael Marletta of the University of California, Berkeley. But Furchgott was right: NO not only controls the dilation of arteries but also performs other key roles. Furchgott shared the 1996 Albert Lasker Basic Medical Research Award with pharmacologist Ferid Murad, then at the Molecular Geriatrics Corporation in Illinois, who also had zeroed in on NO and helped elucidate how it works in the body.
NO is just one of the chemical signals wending through the body, controlling and coordinating cell functions. More than a dozen researchers have won the Lasker Award for determining how those cellular communication channels operate. This article is the first of two delving into the work of Lasker recipients who deciphered new mechanisms of cell signaling.
Taking NO for an Answer
Although NO seems an unlikely signaling molecule, it has several advantageous qualities for communication. “It’s tiny, and it moves very fast,” says physical biochemist Jack Lancaster of the University of Pittsburgh. As a result, “any cell that makes NO can affect hundreds of cells around it,” he says. Still, the molecule is reactive and destructive. “NO can get into all sorts of trouble in a cell,” Marletta says.
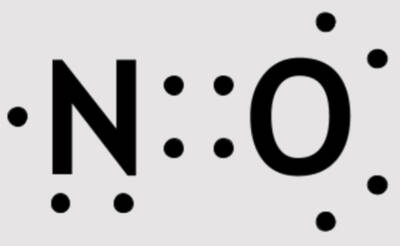
Nitric Oxide Lewis structure
Courtesy of Bioika0101
Furchgott and his team chanced on NO’s role while troubleshooting their experiments on arterial contraction. When slicing out arterial pieces for testing, the researchers had inadvertently been scraping off the innermost cells, known as the endothelial layer. During further experiments in which the layer was in place, acetylcholine—the chemical that had spurred contraction in their earlier studies—triggered the arterial pieces to relax. Furchgott and his team deduced that the endothelial layer was releasing a substance he called the endothelium-derived relaxing factor (EDRF) that loosened the muscles in the arterial wall. But when Furchgott proposed that hypothesis in 1980, he didn’t know what EDRF was.
Working independently, Murad had already gathered evidence pointing to NO. His lab had been probing a messenger molecule within cells called cyclic GMP that orchestrates a variety of responses, including relaxation of blood vessels. In a 1977 study, he and his team showed that several kinds of nitrogen-containing molecules, including NO, could switch on an enzyme that makes cyclic GMP.
In later years, researchers continued to amass evidence for NO’s role, but Furchgott and another scientist, Louis Ignarro of the University of California, Los Angeles, did not publicly claim that the gas was EDRF until 1986. Even then, that was a bold step, Lancaster says. “They made a proposal that I as a biochemist wouldn’t have had the guts to make.”
The discovery that cells were conversing by using a poisonous gas forced scientists to rethink their ideas about cellular communication. The finding also sparked a rush to harness NO for treatments, which led to sildenafil (Viagra), one of biggest-selling drugs in history.
Murad and Furchgott “were superb experimentalists,” Lancaster says. But they also uncovered this unexpected communication channel because
“they were open to interpretation of the data as it spoke to them.”
Those qualities earned them the Lasker Award and the 1998 Nobel Prize in physiology or medicine, which they shared with Ignarro.
Psst, Pass It On
The signals that cells dispatch are useless—and can be harmful—unless other cells respond appropriately. Two researchers who shared the 1989 Albert Lasker Basic Medical Research Award, Michael Berridge and Alfred Gilman, uncovered key mechanisms that allow cells to translate signals into the correct actions.
Berridge, then at Cambridge University in the UK, owed his discoveries partly to an “interest in fly spittle,” as a colleague put it. The flies in question were blowflies, and Berridge dissected out their salivary glands to test how specific chemical messengers trigger saliva production. To keep the juices flowing, he and colleagues found, cells in the glands required calcium, which they often absorbed from their surroundings. But the cells also could dole out calcium they had stashed internally. Using fly and cancer cells, in 1983 Berridge and his team pinned down a molecule, known as inositol trisphosphate (IP3), that allows cells to dip into that stored calcium. The discovery’s implications went far beyond drooling flies because calcium signaling orchestrates a range of functions, including muscle contraction, cell movement, transmission of nerve impulses, and gene expression.
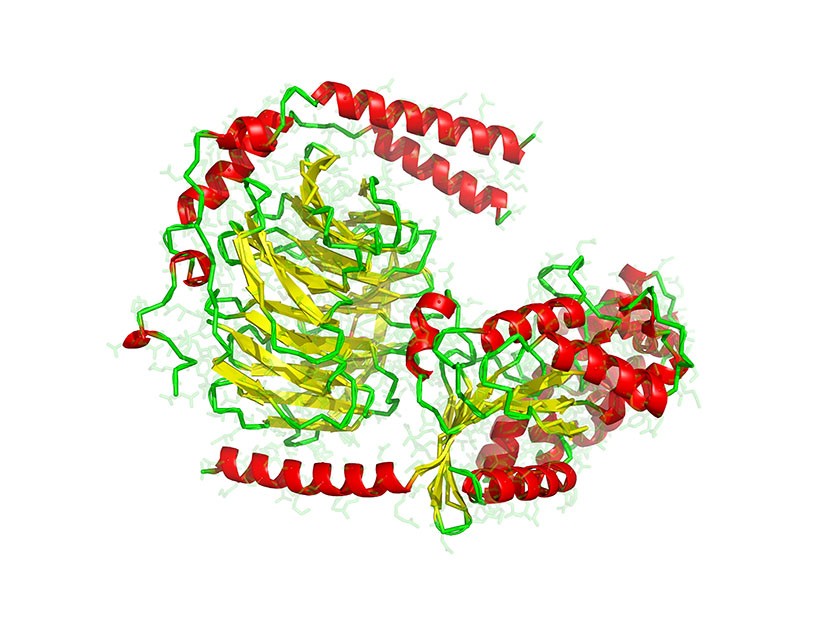
Computer model showing the secondary structure of a heterotrimeric G protein complex. Alpha-helices are red and beta sheets are yellow. G proteins, or guanine nucleotide binding proteins, are molecular switches involved in signal transduction.
Courtesy of Laguna Design / Science Source
Gilman, of the University of Texas Southwestern Medical Center in Dallas, uncovered a class of proteins, the G proteins, that forward signals arriving at a cell’s membrane. Previous studies had shown that when certain hormones bind to a receptor in the membrane, an enzyme in the cell produces a specific messenger molecule that triggers various responses. But researchers didn’t know what turned on the enzyme. Using cancer cells in which that signaling pathway was faulty, Gilman showed in the late 1970s and early 1980s that G proteins were responsible.
In 1994 he shared the Nobel Prize in physiology or medicine for the discovery. G proteins partner with some of the most important receptors in the body, the G protein–coupled receptors. That group includes receptors that capture light in our eyes, detect many smells and tastes, and respond to hormones such as adrenaline.
Hormones Go Nuclear
Like would-be club-goers turned away by a bouncer, many signaling molecules, including insulin, never enter their target cells. But cells admit some of them. The question that vexed researchers was how those molecules worked once inside cells.
In 2004, Pierre Chambon, Ronald Evans, and Elwood Jensen shared the Albert Lasker Basic Medical Research Award for unraveling the intricate mechanism that allows hormones such as testosterone, estrogen, and cortisol—as well as other signaling molecules like vitamins A and D—to exert their effects. Those messengers interact with members of the same family of receptors, known as nuclear receptors, that “touch all aspects of basic physiology,” says Daniel Frigo, a molecular endocrinologist at the University of Texas MD Anderson Cancer Center in Houston.
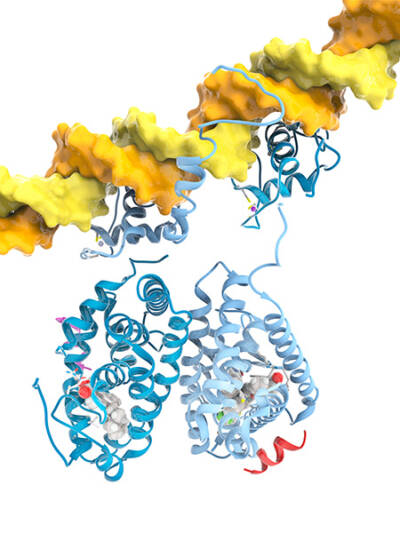
Molecular model of a strand of DNA (yellow) and two nuclear receptors. At left is a retinoic X receptor (blue) in a complex with retinoic acid. At right is a liver X receptor (pale blue) in complex with a drug. Both receptors can bind to DNA specific sequences to act like master gene regulators.
Courtesy of Ramon Andrade 3DCiencia/ Science Source
When Jensen started his research in the mid-1950s, scientists knew that hormones such as estrogen were crossing the cell membrane. But researchers assumed that the molecules were then chemically altered and collaborated with enzymes to produce responses. Jensen, at the University of Chicago, overturned that hypothesis with experiments in which he tracked down minute amounts of radioactively labeled estrogen injected into rats. He showed that the estrogen remained intact and gathered in target organs such as the uterus. Jensen inferred that the hormone was latching onto a receptor there, a hypothesis that further research confirmed. His findings “were eye-opening and set the stage for a later series of discoveries,” says endocrinologist Mitchell Lazar of the University of Pennsylvania.
Starting in the 1980s, Evans and Chambon revealed surprising relationships among receptors targeted by many hormones and other signaling molecules. Evans, then in La Jolla, California, and colleagues identified the gene for the glucocorticoid receptor, which responds to hormones such as cortisol.
Chambon, a molecular biologist at the Institute for Genetics and Molecular and Cellular Biology in Strasbourg, France, and his team pinpointed the gene for one of the estrogen receptors. Both genes, it turned out, resembled the gene that coded for the thyroid hormone receptor—an unexpected kinship. And when Chambon’s and Evans’s groups independently isolated the gene for the receptor that responds to a form of vitamin A, that gene also was a family member.
That clan of nuclear receptors has expanded to 48 molecules in humans. Those receptors help orchestrate key processes such as embryonic development, metabolism, and reproduction. Moreover, about 15% of all drugs target pathways involving nuclear receptors, Lazar says, another indication of their significance. Jensen, Chambon, and Evans had a large impact on their field because of their scientific skill, Frigo says. Jensen “was a superb biochemist,” he says, while Chambon and Evans were virtuosos in the burgeoning field of molecular biology.
“They were so good that oftentimes they were ahead of the curve,”
Frigo says.
The next article will probe the work of seven more Lasker-winning scientists who uncovered signaling mechanisms that allow cells to purge damaging and potentially dangerous proteins, turn on key molecular switches, and even control whether they live or die.
By Mitchell Leslie