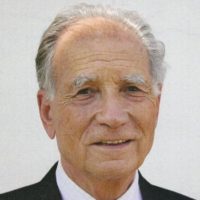
Pierre Chambon
Institute of Genetics and Molecular Cellular Biology
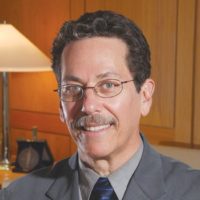
Ronald Evans
The Salk Institute for Biological Studies
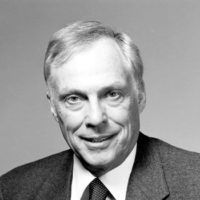
Elwood Jensen
University of Chicago, University of Cincinnati College of Medicine
For the discovery of the superfamily of nuclear hormone receptors and elucidation of a unifying mechanism that regulates embryonic development and diverse metabolic pathways.
The 2004 Albert Lasker Award for Basic Medical Research honors three scientists who opened up the field of nuclear hormone receptor research. Their work elucidated the unexpected common mechanism by which a diverse group of signaling molecules – steroid hormones, thyroid hormone, and fat-soluble molecules such as Vitamin A and D — regulate a plethora of physiological pathways that operate from embryonic growth through adulthood.
A new model for hormone action
Hormones control a vast array of biological processes, including embryonic development, growth rate, and body weight. Scientists had known since the early 1900s that tiny hormone doses dramatically alter physiology, but they had no idea that these signaling molecules did so by prodding genes. The 1950s, when Elwood Jensen began his work, was the great era of enzymology. Conventional wisdom held that estradiol — the female sex hormone that instigates growth of immature reproductive tissue such as the uterus — entered the cell and underwent a series of chemical reactions that produced a particular compound as a byproduct. This compound — NADPH — is essential for many enzymes' operations, but its small quantities normally limit their productivity. A spike in NADPH concentrations would stimulate growth or other activities by unleashing the enzymes, the reasoning went.
In 1956, Jensen (at the University of Chicago) decided to scrutinize what happened to estradiol within its target tissues, but he had a problem: The hormone is physiologically active in minute quantities, so he needed an extremely sensitive way to track it. He devised an apparatus that tagged it with tritium — a radioactive form of hydrogen — at an efficiency level that had not previously been achieved. This innovation allowed him to detect a trillionth of a gram of estradiol.
When he injected this radioactive substance into immature rats, he noticed that most tissues — skeletal muscle, kidneys and liver, for example — started expelling it within 15 minutes. In contrast, tissues known to respond to the hormone — those of the reproductive tract — held onto it tightly. Furthermore, the hormone showed up in the nuclei of cells, where genes reside. Something there was apparently grabbing the estradiol.
Jensen subsequently showed that his radioactive hormone remained chemically unchanged once inside the cell. Estrogen did not act by being metabolized and producing NADPH, but presumably by performing some job in the nucleus. Subsequent work by Jensen and Jack Gorski established that estradiol converts a protein in the cytoplasm, its receptor, into a form that can migrate to the nucleus, embrace DNA, and turn on specific genes.
From 1962 to 1980, molecular endocrinologists built on Jensen's work to discover the receptors for the other major steroid hormones — testosterone, progesterone, glucocorticoids, aldosterone, and the steroid-like vitamin D. In addition to Jensen and Gorski, many scientists — notably Bert O'Malley, Jan-Ake Gustafsson, Keith Yamamoto, and the late Gordon Tompkins — made crucial observations during the early days of steroid receptor research.
Clinical applications of estrogen-receptor detection
Clinicians knew that removing the ovaries or adrenal glands of women with breast cancer would stop tumor growth in one out of three patients, but the molecular basis for this phenomenon was mysterious. Jensen showed that breast cancers with low estrogen-receptor content do not respond to surgical treatment. Receptor status could therefore indicate who would benefit from the procedure and who should skip an unnecessary operation. In the mid-1970s, Jensen and his colleague Craig Jordan found that women with cancers that contain large amounts of estrogen receptor are also likely to benefit from tamoxifen, an anti-estrogen compound that mimics the effect of removing the ovaries or adrenal glands. The other patients — those with small numbers of receptors — could immediately move on to chemotherapy that might combat their disease rather than waiting months to find out that the tumors were growing despite tamoxifen treatment. By 1980, Jensen's test had become a standard part of care for breast cancer patients.
In the meantime, Jensen set about generating antibodies that bound the receptor — a tool that provided a more reliable way to measure receptor quantities in excised breast tumor specimens. His work has transformed the treatment of breast cancer patients and saves or prolongs more than 100,000 lives annually.
Long-lost relatives
By the early 1980s, interest in molecular endocrinology had shifted toward the rapidly developing area of gene control. Pierre Chambon and Ronald Evans had long wondered how genes turn on and off, and recognized nuclear hormone signaling as the best system for studying regulated gene transcription. They wanted to know exactly how nuclear receptors provoke RNA production in response to steroid hormones. To manipulate and analyze the receptors, they would need to isolate the genes for them.
By late 1985 and early 1986, Evans (at the Salk Institute in La Jolla) and Chambon (at the Institute of Genetics and Molecular and Cellular Biology in Strasbourg, France) had pieced together the glucocorticoid and estrogen receptor genes, respectively. They noticed that the sequences resembled that of v-erbA, a miscreant viral protein that fosters uncontrolled cell growth. This observation raised the possibility that v-erbA and its well-behaved cellular counterpart, c-erbA, would also bind DNA and control gene activity in response to some chemical activator, or ligand. In 1986, Evans and Björn Vennström simultaneously reported that c-erbA was a thyroid hormone receptor that was related to the steroid hormone receptors, thus uniting the fields of thyroid and steroid biology.
Award presentation by Michael Brown
This year's Lasker Basic Research Award honors three brilliant scientists who taught us how cells communicate. To understand their work, we must first appreciate that our bodies are composed of 10 trillion cells. That's 1 with 13 zeroes. If each cell operated as an individual, our bodies would be as chaotic as the US Senate. Fortunately, cells are not senators. They are like musicians playing in a well-conducted orchestra. Each cell has a special task that it performs only when instructed by another cell. How do cells receive these instructions? How do they interpret the instructions so as to perform the right task? The answer was discovered by our three honorees. It is a family of proteins called nuclear receptors — or as George Bush would say, nucular receptors.
Acceptance remarks
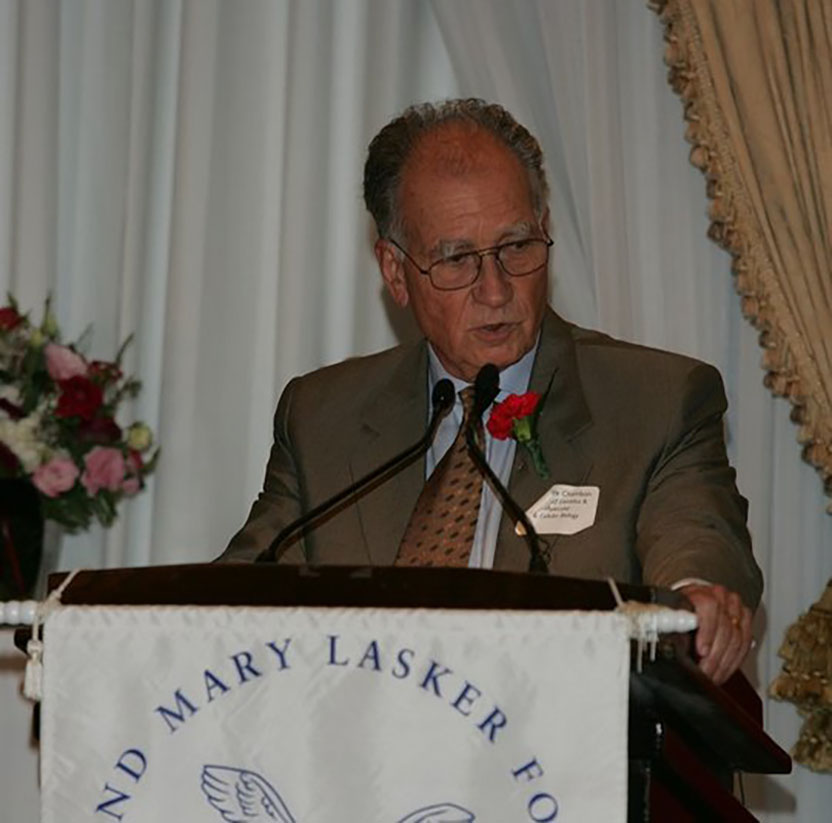
Acceptance remarks, 2004 Lasker Awards Ceremony
In accepting the 2004 Lasker Award in Basic Medical Research, I wish to express my deep gratitude to the Albert and Mary Lasker Foundation and to its chairman (Mr. James W. Fordyce) and president (Dr. Neen Hunt) for this prestigious award and the recognition that it conveys.
I am particularly happy and thankful to the Albert Lasker Medical Awards Jury and to Dr. Joe Goldstein, its chairman, because this year’s Award that I share with Elwood Jensen and Ron Evans nicely illustrates how science can contribute to forge friendships in an atmosphere of fruitful competition.
It is a great honor and privilege to be a recipient of this Award, in a country which has given such a high priority to the development of higher education, science and medicine. I accept it as the representative of the many members of my laboratory, who have worked and collaborated with me over the last 40 years. Our achievements are the fruit of their enthusiasm and devotion to science.
I have attempted, throughout my scientific life, to address basic problems related to the control of expression of genetic information in animals, notably in mammals, that have to be solved in order to advance our understanding of pathological disorders. The work of my laboratory has contributed to the so-called ‘Genetic Revolution’ which, over the last 30 years, has drastically changed our ideas on the structural organization, expression, and evolution of the genetic material. What is the organization of the building blocks of this material that we name genes, how are they turned on and off in our cells during development, and then in adult tissues, how are the different cell types generated, and which role do hormones play in these processes? These are some of the questions that we have addressed. We have uncovered some basic principles, and hopefully, some of our studies are leading, and will further lead, to a better understanding of diseases and to more efficient therapies.
Science is the most challenging of modern adventures, a unique chance to discover the unknown. The excitement of being a molecular geneticist and biologist is to participate to the exploration of life and to discover how it operates. It is also the conviction that progress in biological sciences will ultimately result in a molecular understanding of all diseases, paving the way to their successful treatment. Most importantly, being a molecular geneticist gave me a chance to understand from where we, human beings, originate through 4 billion years of biochemical evolution, and to realize that we are all alike, with just a few small differences that are due to genetic and environmental chance. Clearly, if some of us are smarter, it is not their own merit! What modern biochemistry, molecular biology, and genetics have taught us are lessons of tolerance, humility, and responsibility.
Let me thank again — very sincerely — all of those who enabled me to participate in the adventure of science and to be present here today.
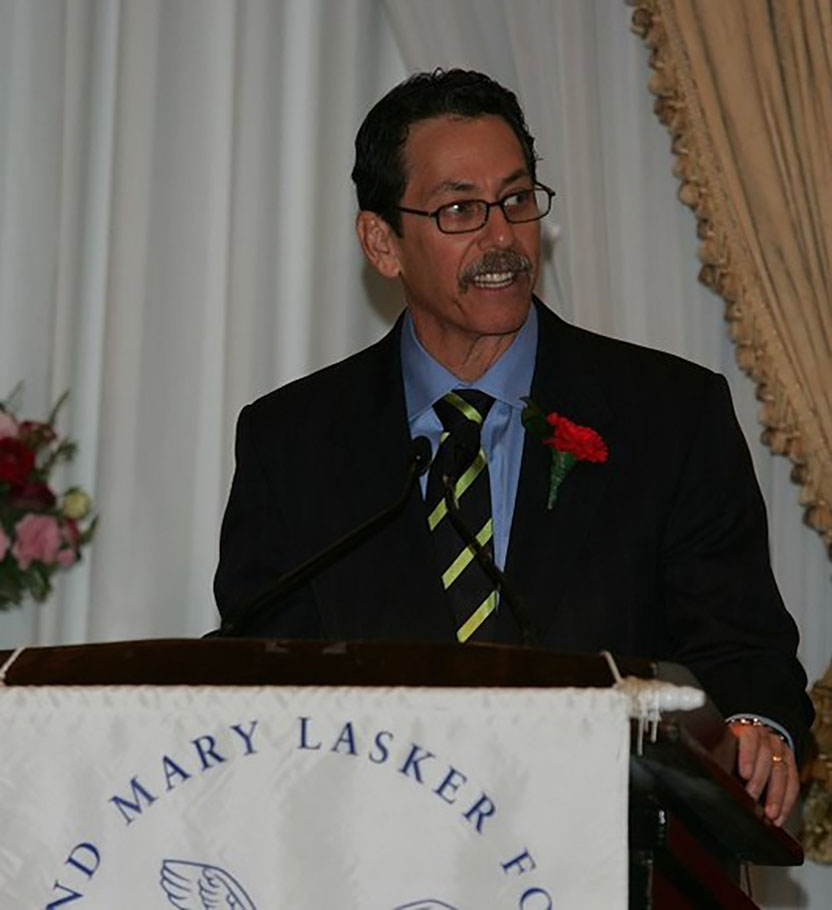
Acceptance remarks, 2004 Lasker Awards Ceremony
It is a great pleasure to be here! I wish to thank Joe for his elegant overview and Mike for your warm and personal comments about our discoveries. I was even beginning to like the guy you were talking about.
I am truly delighted to be the recipient of the 2004 Lasker Award. I want to thank the distinguished jury for this honor. The vetting process is extremely rigorous — I knew this when they asked for dental records. I am greatly honored to be included in the select group of physicians and scientists who have won this prestigious award. I wish to heartily congratulate my co-recipients, Elwood Jensen and Pierre Chambon.
At times it seemed that our work was everything, enveloping us in a surreal world of complex ideas and swirling emotion. It was like we had fallen into that magical tunnel described by Lewis Carroll depositing us at the entrance to Wonderland… an entrance that was sealed and could be unlocked only by asking the right question.
Science is about asking the right question — as I tell every one who joins my lab, “if you want a big answer you must ask a big question.” I have had the good fortune to work with a remarkable cadre of people, my “comrades in science,” some of whom are sitting at my table, who were not afraid ask and work on the big questions.
The idea of asking questions takes me back to the time I first met Francis Crick. It was said Francis’ great gift was asking the right question. I remember at Salk when I first went to his office to discuss my work. An office first occupied by the famed Jacob Bronowski with a framed picture of Charles Darwin adorning the wall, with Francis sitting in Shakespearean mode at his desk, I began to wonder “Lord, why am I here? And how can I get out?” Those were my first questions. Realizing I was nervous, Francis asked his first of many questions: “Ron, would you like some cinnamon toast? And perhaps some tea?” “Kathleen,” he said, “some cinnamon toast and tea for Ron.” Deceptively simple, but it settled me down and was a brilliant start to a 26-year-long relationship.
So what are the next questions? For us, we ask how our studies of transcriptional physiology can help to understand human development and control human disease. Biomedical research can only be pushed forward by the next generation and it is my hope to encourage the spirit of young people to pursue science and bring their energy and enthusiasm to help answers to these questions.
Finally, in addition to the idealistic aspects, science is a grueling and competitive discipline and I want to thank my wife, Ellen, for unflagging emotional support, advice and love. I also acknowledge my daughter, Lena — as of three weeks ago a freshman at Columbia — who spackles me with style and gives me perspective on what life is all about.
My thanks to you all for sharing this special day.
I end on the note that this week in 1687, Sir Isaac Newton published his theories of gravitation in the “Principles” — so all of you may be comforted by the knowledge that what stood up will now sit down.
Thank you.
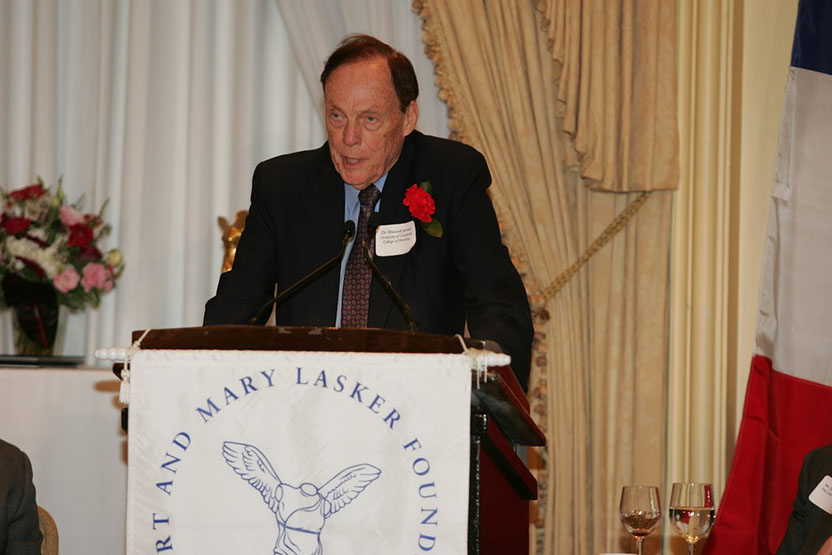
Acceptance remarks, 2004 Lasker Awards Ceremony
First, I can say that I am thrilled to be here, not only by the enjoyment of this festive occasion but also by the opportunity to join the impressive group of scientists who have received Lasker Awards in the past. When I was growing up, one of the adages to which we were exposed was “you are known by the company you keep.” When one surveys the amazing list of Lasker Laureates, one must agree that, at least by this criterion, the five scientists who are being honored today seem to have heeded this admonition.
To have the opportunity, as well as the obligation, to sit down and reflect on how we came to do the research being recognized today is of immense value in planning future activities. As explained in more detail in my article entitled “From Chemical Warfare to Breast Cancer Management” in the October issue of Nature Medicine, of which you have received a copy, my path was rather indirect. Having majored in chemistry at Wittenberg College in Ohio, I had already begun graduate training in organic chemistry at the University of Chicago when World War II came along. Although I had a private pilot license and wanted to join the Air Force, they would not accept contact lenses, so I spent the war on a project in the Department of Chemistry, synthesizing new and more effective poison gases while finishing my graduate studies and flying for the Civil Air Patrol on the weekends. Many of these toxic agents were quite formidable and actually put me in the hospital on two occasions, so I was glad that we did not need to drop them on the baby in Göttingen who 40 years later was to become my wife. So Peggy can be here today to help us celebrate.
The poison gas studies stimulated my interest in biology and medicine. But when the war was over, I had a family and was a bit too old to begin four years of medical school. So I had to settle for a one-year Guggenheim Fellowship to learn steroid hormone chemistry at the Swiss Federal Institute of Technology in Zürich. While in Switzerland, I had the opportunity to climb the Matterhorn, which, as a novice, was the most difficult physical challenge of my life. More important, I learned the concept of ‘alternative approach’ from the fact that the Matterhorn was the last major peak in Europe to be climbed, simply because people had always tried it from the Italian side, which looks much friendlier than the sheer northeast face usually seen in photographs. An Englishman named Edward Whymper decided to test the “impossible” northeast face, found it relatively easy, and, in July 1865, he and his party were the first to reach the top. As explained in the Nature Medicine article, two of our most important contributions, discovery of steroid hormone receptors and preparation of the first antibodies to them, depended on approaches different from what other laboratories were using.
Finally, during the past few weeks we have had a wonderful opportunity to learn more about the Lasker Foundation and what they have done and continue to do in supporting and stimulating biomedical research. This is an inspiration to all of us, as well as a cause for strong optimism for the future.