by Joseph L. Goldstein
The creative process in art and science involves two phases—generating and exploring new and wild ideas (building castles in the sky) and then narrowing down and focusing on the most tractable and useful ones (building houses of cards that don’t topple over).
In 1927, the theoretical physicist Paul Dirac, who was 25 years old at the time, published a mathematical equation that predicted the existence of a new atomic particle with the same mass as the electron but with the opposite charge. Such a particle, called the positron, was discovered experimentally four years later in cosmic rays, confirming Dirac’s theory (Rovelli, 2021).
Dirac’s equation not only did its intended job of describing the behavior of electrons, but it also predicted the first glimpse of antimatter, which we now know to be composed of many types of subatomic particles that make up as much as half of the universe (Farmelo, 2009). The Dirac equation has been referred to as “a piece of magic” (Wilczek, 2003) and “the most seductive equation in science” (Overbye, 2002), owing to the way in which reality and truth are revealed in a compressed and beautifully poetic way.
Dirac’s Big Idea: How Imagination Leads to New Ideas
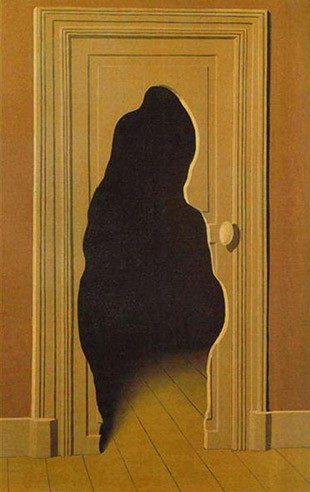
Figure 1. The Unexpected Answer
René Magritte (1933). Oil on canvas. 32.3 x 21.4 in. ©2022 C. Herscovici/Artists Rights Society (ARS), New York.
When Dirac was asked where he gets his ideas, his reply was pithy and to the point – ideas come from the imagination. His exact words were: “You just have to try and imagine” (Judson, 1987).
So, how does a scientist get new ideas by just “trying and imagining”? Imagination is one of those elusive concepts that defies a concrete definition. At its simplest, imagination allows one to explore things, real or unreal, that are generated from within and are not perceived from the present environment or outside world. In this sense, the ideas that emerge from one’s imagination are thus inventions of the human mind.
Magritte and the Mystery of the Mind: Building Castles in the Sky
The artist who, in my view, best exemplifies the inventiveness of the human mind is the surrealist painter Rene Magritte (1898-1967). Magritte was fond of saying that the mind sees in two different senses: it sees, as with the eyes, and it sees a question with no eyes (Gablik, 1976). For Magritte, imagination was a way to use painting to evoke a thought that transcends one’s knowledge of the current world, which would then allow the emergence of a magical new insight.
Magritte’s painting The Unexpected Answer illustrates the mystery of the imagination (Figure 1). The cutout of the closed door allows a glimpse and passage into an unknown world—or in the Dirac sense into the surreal world of the imagination. Another Magritte painting, The Castle in the Pyrenees (also referred to as The Castle in the Sky), hints at what one might find behind the door. In this painting, Magritte depicts a massive rock suspended in midair above an ocean (Figure 2). The magical quality of the rock is emphasized by the realism of the waves beneath it. On top of the rock is a castle with fortified walls.
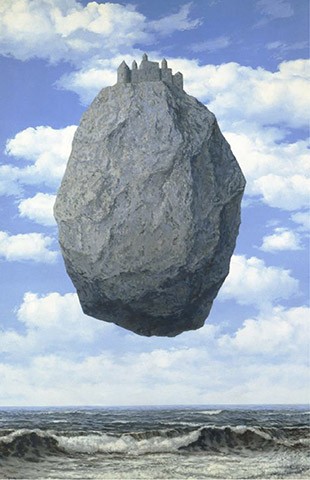
Figure 2. The Castle of the Pyrenees
René Magritte (1959). Oil on canvas. 78.7 x 57.1 in. Israel Museum. ©2022 C. Herscovici/Artists Rights Society (ARS) New York.
The heavy rock, which should fall into the ocean, is shown as a buoyant object, which defies the laws of physics and is impossible in real life. Nonetheless, the uplifting view of the castle in the sky evokes a feeling of wonder—a feeling that allows one to leap into the unknown where all sorts of ideas can be imagined. The expression “castles in the sky” is a widely used metaphor for one’s dreams, aspirations, and wild ideas that may seem farfetched and have little chance of success, but seem worth an effort to reach.
The more castles one builds in the sky, the more imagined castles (ideas) will emerge. Prominent scientists like the chemist Linus Pauling, the immunologist Peter Medawar, and the molecular biologist François Jacob have told us that their best ideas came when they played like children who have infinite curiosity and little fear of taking risks. Letting their minds misbehave set off sparks to creative thinking, flooding their brains with multiple ideas—most were bad, but a few good ones surfaced (Marinacci, 1995; Medawar, 1982; Jacob, 1988).
Jean-Siméon Chardin: How to Build a House of Cards that Doesn’t Topple Over
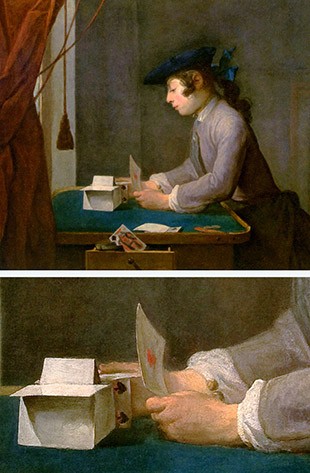
Figure 3. Boy Building a House of Cards
Jean-Siméon Chardin (1735). Oil on canvas. 32 x 40 in. Rothschild Collection, Waddesdon Manor, Buckinghamshire, England.
Once scientists come up with grand castles of ideas, they face the challenge of building their castles or realizing their ideas in such a way that they do not collapse and fall to the ground like a house of cards. This is where the work of the 18th century French artist Jean-Siméon Chardin (1699-1779) can be instructive to scientists. One of the most revered of French painters, Chardin was a master of intimate genre scenes in which he captured on the canvas one or two figures from the Parisian middle class engaged in work or play (Prigent and Rosenberg, 2000). Among his most admired works is a suite of four paintings entitled House of Cards, which were painted from 1735 to 1737 (Carey, 2012). In the first of the four paintings, a young boy is shown deeply absorbed in the tricky art of adding the Ace of Hearts to a fragile castle-like structure constructed from four or five other cards (Figure 3). If the placement of the Ace card is not balanced perfectly, the whole structure topples over with all the cards falling flat on the table.
Figure 4 shows a composite of the four versions of Chardin’s House of Cards, each depicting a different boy concentrating intensely on the task in hand. The expanded view of the playing cards at the bottom of Figure 4 illustrates Chardin’s skill in manipulating objects in painted space. He creates a virtual balancing act on the canvas by depicting the cards from multiple angles—stacked, side-on, folded, and leaning next to one another.
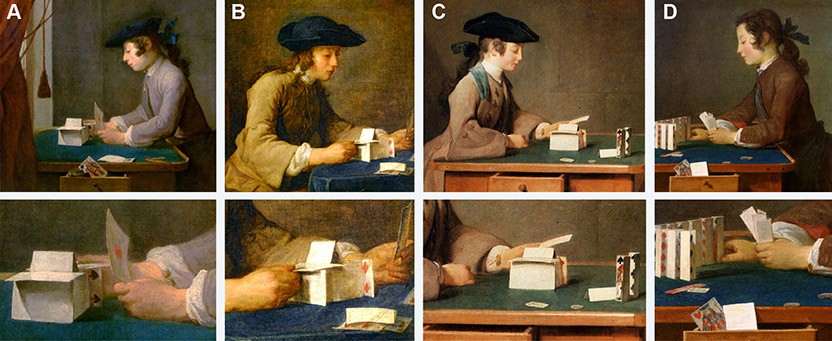
Figure 4. Composite Image of Chardin’s Four Versions of The House of Cards
(A) Boy Building a House of Cards (1735). Same painting as in Figure 3.
(B) The House of Cards (1736-37). Oil on canvas. 30 x 27 in. Musée du Louvre, Paris.
(C) The Son of Monsieur Le Noir amusing Himself in making a House of Cards (1736-37). Oil on canvas. 24 x 28 in. National Gallery, London.
(D) Young Man amusing Himself with Cards (1737). National Gallery of Art, Washington, D.C.
Carrying out scientific experiments, like balancing cards, requires not only technical dexterity, but also passion, determination, and persistence. Maintaining a strong foundation that prevents collapse of the cards (and the experiments) also requires experimental finesse, which can be defined as the ability to spot a discrepancy quickly so that one knows when you have a finding that doesn’t fit. This ability to rapidly imagine all possibilities, good or bad, is a core characteristic of scientific creativity. Completing a successful experiment from an original idea is like building a house of cards that does not fall to the ground.
Richard Serra’s House of Cards: From a Virtual to a Real Balancing Act
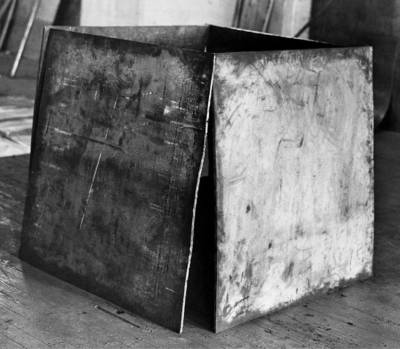
Figure 5. One Ton Prop (House of Cards)
Richard Serra (1969). Lead antimony, four plates. Each plate 48 x 48 x 1 in. Museum of Modern Art, New York. ©2022 Richard Serra/Artists Rights Society (ARS), New York.
In 1967, 234 years after Chardin created a virtual balancing act in his House of Cards paintings, the American sculptor Richard Serra (born, 1939) produced a real balancing act in a monumental sculpture entitled One Ton Prop (House of Cards). Serra’s sculpture is made up of four square lead plates with one edge of each lead “card” resting against each other to form an open cube-like structure (Figure 5). Each of the lead plates is 4×4 feet in size, and together the four plates weigh one ton. Yet, paradoxically, the massive four lead slabs have a weightless quality and exude an elegance of simplicity.
The most innovative aspect of Serra’s sculpture is that the shape of the cube appears freestanding without any welding or extraneous support to balance the individual pieces of lead, which contact each other only at their upper corners. To achieve such a delicate balance, Serra meticulously positioned each lead “card” in such a way that the weight and gravity of the individual “cards” counterbalance each other (McShine and Cooke, 2007).
How Making a List of Verbs Inspired Serra’s Sculptures
The inspiration for One Ton Prop (House of Cards) did not come from Chardin’s 18th century paintings. Rather, it began in the early 1960s when Serra compiled a list of about 200 verbs like “to roll, to bend, to prop, to balance, to swivel, etc.” Serra undertook this verbing exercise to enumerate the physical processes involved in creating a sculpture (Serra and Weyergraf, 1980). This “Castle in the Sky” approach allowed him to move beyond traditions of conventional sculptures that involved modeling, casting, molding, pedestals, etc. To this point, Serra wrote: “It struck me that instead of thinking what a sculpture is going to be and how you’re going to do it compositionally, what if you just enacted those verbs in relation to a material, and didn’t worry about the results. So I started tearing and cutting and folding lead.” (McShine and Cooke, 2007).
Serra’s Revolutionary Ideas for Transforming Sculpture
In the early 1970s after completing House of Cards, Serra experienced another “Castle in the Sky” moment, leading to a radical change in the style and scale of his work. Unlike traditional sculptures in which figures are rendered in a 3-dimensional form, Serra’s new approach involved the construction of giant environmental sculptures that would promote direct interactions with the viewer, who must walk through the work in order to understand and appreciate its beauty.
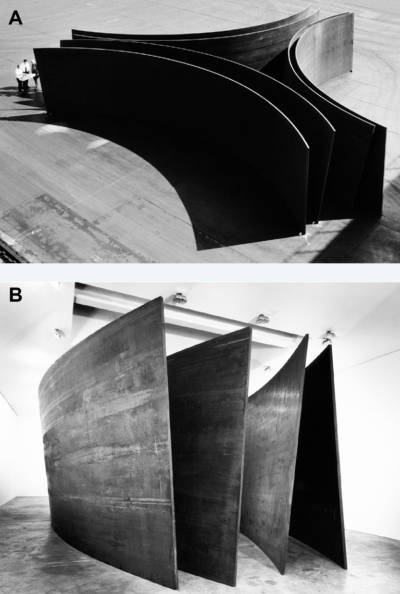
Figure 6. Two Immense “Walk-in” Sculptures
(A) Switch. Richard Serra (1999). Weatherproof steel, six plates. Each plate 13 ½ ft. high x 52 ft. along the card x 2 in, thick. Museum of Modern Art, New York ©2022. Richard Serra/Artists Rights Society (ARS), New York.
(B) Intersection II. Richard Serra (1992). Weatherproof steel, four identical conical sections. Two sections 14 ft. high x 51¾ ft. along the chord x 2.1 ft. thick. Museum of Modern Art, New York. ©2022 Richard Serra/Artists Rights Society (ARS), New York.
Two examples of Serra “walk-in” sculptures are illustrated in Figure 6. The sculpture Switch is made up of six identical steel plates; each weighs 28 tons and is more than 13-feet high and 50-feet long (Figure 6A). The six plates are arranged in three pairs, each formed in the shape of a curving arc. Viewers can wander around, into, and through the curves and winding passages and many get lost within the swooping structure. The sculpture shown in Figure 6B, Intersection, is another example of a monumental Serra “walk-in” with seductive curves, angles, and inverted shapes that tease the eye and the mind.
Imagination, Ideas, and Focus: Keys to Scientific Experiments that Stand the Test of Time
As exemplified by the modus operandi of Dirac, Magritte, Chardin, and Serra, success involves having the imagination to come up with new ideas and the skill to narrow down and focus on those ideas that can be elaborated in such a way that the results do not collapse like a house of cards. Like a badly placed card, an experiment built on a fragile idea will rest on such shaky grounds that its results will crumble. Chardin’s and Serra’s House of Cards capture many of the key requirements for building a strong foundation for one’s ideas – namely, technical expertise, passionate persistence, and unwavering determination.
2022 Lasker Awards: Building ‘Castles in the Sky’ and ‘Houses of Cards’ that have Stood the Test of Time
The discoveries made by this year’s Lasker Awardees began as ‘Castles in the Sky,’ and advanced to ‘Building Houses of Cards’ that have stood the test of time, as described below.
Basic Award. The 2022 Lasker Basic Research Award honors three scientists for discoveries concerning the integrins—key mediators of cell-matrix and cell-cell adhesion in physiology and disease. The awardees are Richard O. Hynes (Massachusetts Institute of Technology), Erkki Ruoslahti (Burnham Prebys Medical Discovery Institute, La Jolla), and Timothy A. Springer (Boston Children’s Hospital/Harvard Medical School).
In the mid 1970’s, Hynes and Ruoslahti independently focused on an extracellular matrix (EMC) protein, now called fibronectin, which they later showed to be a ligand for several integrin receptors involved in cell-matrix adhesions. At roughly the same time, Springer discovered a family of three leukocyte membrane proteins that he later showed to be the first integrin receptors involved in cell-cell adhesions in the immune system. The research of these three scientists paved the way for our current understanding of the integrins as a conserved family of transmembrane receptors that facilitate dynamic adhesive events between cells and the ECM and between different types of cells. These adhesive events play essential functions in a diverse range of biological actions, involving formation of the ECM and its linkage with the intracellular actin cytoskeleton, migration of neural crest cells during embryogenesis, platelet aggregation and clot formation, immune cell recruitment, cell growth and metastasis, killing of virus-infected cells, and clearance of bacteria and other pathogens.
An early breakthrough in cell-matrix adhesion was Ruoslahti’s discovery in 1984 that fibronectin contained within its 1008-amino acid sequence a tripeptide motif RGD (Arg-Gly-Asp) that served as a recognition signal for cell adhesion. Ruoslahti also found that seven other ECM proteins, including vitronectin, fibrinogen, von Willebrand factor, and laminin, contained the same RGD motif. This discovery provided a suite of RGD-containing matrix ligands that facilitated the identification and purification of the first cell-matrix integrin receptors by Ruoslahti, Hynes, and others.
The first breakthrough in cell-cell adhesion came about in the late 1970s when Springer performed a screen for monoclonal antibodies that would block lymphocyte functions. The result was the initial discovery of LFA-1 (for Lymphocyte Function Antigen-1), a transmembrane protein on the surface of lymphocytes and other leukocytes. Later work showed that LFA-1 belongs to a family with two other leukocyte receptors, Mac-1 and p150,95. Each of them – LFA-1, Mac-1 and p150,95 – is a heterodimer with an identical β-subunit, but with a distinct α-subunit. Together, the three proteins play essential roles not only in lymphocyte function but also in leukocyte chemotaxis and phagocytosis of invading bacteria. This latter action is achieved by their adhesion to the ICAM transmembrane proteins on activated endothelial cells, which leads to emigration of the leukocytes from the bloodstream to sites of inflammation. Inherited mutations that disrupt the common β-subunit of the three leukocyte integrins impair their phagocytosis of bacteria, producing an inherited human disease called Leukocyte Adhesion Deficiency, characterized by recurrent bacterial infections. That this disease is caused by mutations in the shared β-subunit provided strong support for Springer’s concept of cellular adhesion reactions in inflammation and host defense.
The year 1987 was a pivotal year in the evolution of the integrin field. By that time, it had become clear from the cDNA sequencing work of Springer, Ruoslahti, and Hynes that three functionally unrelated classes of adhesion receptors—the leukocyte surface receptors LFA-1, Mac-1, and p150,95; the ECM adhesion receptors for fibronectin and vitronectin; and the platelet GP IIb-IIIa receptor—were all related structurally. Their deduced protein sequences revealed the surprising finding that the receptors for these three totally different physiological systems were noncovalent heterodimers consisting of homologous α and β-subunits. This was the breakthrough that propelled the field of integrin research.
In a prescient Cell review entitled “Integrins: A Family of Cell Surface Receptors,” Hynes (1987) captured the excitement of what was to become a robust new field. When this review was written, 11 integrins had been described, only 6 of which had a known ligand and function. One of the most functionally characterized integrins at the time of the review was the platelet GP IIb-IIIa receptor, which consists of the subunits α11β and β3. Originally defined biochemically in the early 1980s by David R. Phillips (Gladstone Institute), GP IIb-IIIa plays a key role in platelet aggregation and clot formation. In response to platelet stimulation by agonists such as thrombin, the activated GP IIb-IIIa stops bleeding at sites of vascular injury by binding to several RGD-containing components of the ECM, including fibrinogen, fibronectin, von Willebrand factor, and vitronectin. These adhesive cell-matrix interactions trigger crosslinking of the platelets into aggregates that form the clot. Mutations that disrupt either the α11β subunit or the β3 subunit of the platelet GP IIb-IIIa integrin produce an inherited human bleeding disorder called Glanzmann thrombasthenia.
It is now known that the integrin family consists of 24 distinct heterodimeric receptors, each containing one α-subunit and one β-subunit. In mammals, there are 18 different α-subunits and 8 different β-subunits that assemble into the 24 distinct receptors. Although each of the integrin receptors is responsible for a different physiological function, the common feature of all 24 receptors is their role in cell-matrix or cell-cell adhesions.
Five FDA-approved, integrin-targeting drugs are currently available. One is the monoclonal antibody Vedolizumab (Entyvio®), which targets cell-cell interactions by preventing T cells from homing to the gut. It is used in treatment of ulcerative colitis and Crohn’s disease. The drug Lifitegrast (Xiidra®) is a small molecule antagonist of LFA-1 that prevents lymphocyte adhesion, thereby reducing T cell-mediated inflammation. It is administered as eyedrops to treat dry eye disease. Xiidra owes its conceptual origin to Springer’s original work on leukocyte integrins.
Integrin research continues as one of the most active fields in biomedical science today. Since 1987, more than 80,500 integrin-related publications have appeared in the literature. A remarkable aspect of our three Lasker awardees—Hynes, Ruoslahti, and Springer—is that they not only started the integrin field nearly 50 years ago, but they have each remained active contributors to this day.
Clinical Award. The 2022 Lasker-DeBakey Clinical Award is given for the discovery of fetal DNA in maternal blood, leading to noninvasive prenatal testing for Down syndrome. The scientist being honored is Yuk Ming Dennis Lo from The Chinese University of Hong Kong.
Trisomy 21, the cause of Down syndrome, is the most common chromosomal disorder throughout the world. Each year about 1 in 800 babies in the US are born with Down syndrome. The risk for Down syndrome increases dramatically with the age of the mother—from 1 in 1340 for a 25-year-old mother to 1 in 350 at age 36 to 1 in 85 at age 40. Prior to 2011, the prenatal diagnosis of Down syndrome could be done only by amniocentesis and chorionic villus biopsy – two procedures with a 0.5 to 1% damage to the fetus.
In 1997, Lo discovered the presence of cell-free fetal DNA in the plasma of pregnant women. This was the breakthrough that opened a new approach for prenatal diagnosis of Down syndrome and other chromosomal diseases. Over the next 10 years, Lo elucidated the fundamental characteristics of this newfound biological phenomenon, including determining the gestational-age variation in appearance of fetal DNA in maternal plasma.
In 2007, Lo published an approach for detection of Down syndrome based on a digital PCR molecular counting method that was sensitive, but not practical. One year later in 2008, he demonstrated that the most efficient and accurate method for diagnosis was achieved with massively parallel genomic DNA sequencing of maternal blood, which could be used to quantify and “count” the amount of chromosome 21 sequences. Similar results were published independently and contemporaneously by Stephen Quake (Stanford University).
Using his counting method, Lo carried out the first large-scale clinical validation of this approach, culminating in 2011 in the commercial launch of prenatal tests for Down syndrome. Since its introduction 11 years ago, noninvasive prenatal testing for Down syndrome has become available in more than 60 countries. More than 10 million tests are performed each year. In addition to prenatal diagnosis, Lo’s discovery has opened the door to a wide range of applications including the use of cell-free DNA for early cancer screening and for following the course of cancers during and after therapy.
What is especially impressive about Lo’s story is his assiduous pursuit from the initial discovery of cell-free DNA in maternal plasma (1997) to its clinical application (2011). Many scientists contributed along the way, but Lo was the only one who was present from beginning to end.
For a detailed account of the discoveries of this year’s Lasker Awards, please refer to the Lasker website and to the articles in this issue of Cell.
References
Carey, J. (2012). Taking Time: Chardin’s Boy Building a House of Cards and Other Paintings. (London: Paul Holberton Publishing).
Farmelo, G. (2009). The Strangest Man: The Hidden Life of Paul Dirac, Quantum Genius. (London: Faber and Faber, Ltd.).
Gablik, S. (1976). Magritte. (Boston: New York Graphic Society).
Hynes, R.O. (1987). Integrins: A family of cell surface receptors. Cell 48, 549-554.
Jacob, F. (1988). The Statue Within: An Autobiography. (Plainview, NJ: Cold Spring Harbor Laboratory Press).
Judson, H.F. (1987). The Search for Solutions. (Baltimore: Johns Hopkins University Press).
Marinacci, B. (1995). Linus Pauling in His Own Words (New York: Simon & Schuster, Inc.).
McShine, K. and Cooke, L. (2007). Richard Serra Sculpture: Forty Years. (New York, Museum of Modern Art).
Medawar, P. (1982). Pluto’s Republic. (Oxford, UK: Oxford University Press).
Overbye, D. (2002). The most seductive equation in science: Beauty equals truth. New York Times, March 26, 2002.
Prigent, H. and Rosenberg, P. (2000). Chardin: An Intimate Art. (New York, Harry N. Abrams, Inc.).
Rovelli, C. (2021). Helgoland: Making Sense of the Quantum Revolution. (New York: Riverhead Books).
Serra, R. and Weyergraf, C. (1980). Richard Serra: Interviews, Etc. 1970-1980. (Yonkers, NY: Hudson River Museum).
Wilczek, F. (2003). A piece of magic: The Dirac equation. In: It Must Be Beautiful: Great Equations of Modern Science, G. Farmelo, ed. (London, UK: Granta Books), pp. 132-160.