Just before Christmas in 1978, Rod Saunders, a 46-year-old hardware store manager, sat down in a lab at the University of Melbourne in Australia for a hearing test. As an audiologist read a list of common words into a microphone, Saunders repeated the ones he understood in a broad Australian accent. Saunders did not catch all the words, recalls otolaryngologist Graeme Clark, the scientist supervising the test, but “he got a significant number right or nearly right.”
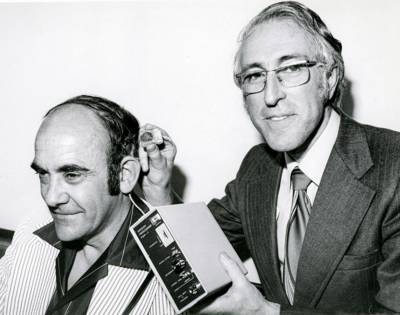
Rod Saunders (left) is wearing a bionic ear designed by Graeme Clark, who holds the processor that transforms speech sounds into electrical stimulation for the inner ear.
Image courtesy of Graeme Clark
That Saunders heard any of the words was impressive because he had been totally deaf for nearly two years after a car accident. He was the first recipient of what Clark and his colleagues called a bionic ear, a device to restore hearing. Four months before the test, Clark had performed a 9-hour operation to implant a tiny electronic receiver underneath the skin behind Saunders’ left ear and then snake a bundle of electrodes from the receiver into the cochlea, the coiled structure in the inner ear that transforms sound waves into nerve impulses the brain can decipher. During the test, Saunders wore behind his ear a small transmitter that was connected to a computer, which broke down speech into electrical signals that stimulate the cochlea. Clark and his team had been developing their bionic ear for nearly a decade and were running out of research money. But that Christmastime test confirmed that they had achieved a medical first, enabling Saunders to regain at least some of his ability to comprehend speech. Clark then did something that’s “not very Australian,” he admits. “I went into the next room and burst into tears of joy.”
Along with Ingeborg Hochmair-Desoyer of the Austrian company MED-EL and Blake Wilson of Duke University, Clark shared the 2013 Lasker Award for developing modern cochlear implants, which have allowed more than 1 million people worldwide to hear again — or, in some cases, for the first time. “It’s the best neuroprosthetic device out there,” says biomedical engineer Gregory Clark (no relation to Graeme) of the University of Utah.
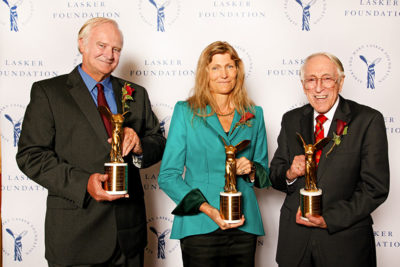
Left to right: 2013 Lasker Award recipients Blake Wilson, Ingeborg Hochmair-Desoyer, and Graeme Clark
Graeme Clark, Hochmair-Desoyer, and Wilson are among more than a dozen scientists who have won a Lasker Award for work in neuroscience. Some of these researchers teased out fundamental mechanisms that enable us to feel, move, think, and remember. Other winners tapped into neuroscience knowledge to develop new methods for treatment or rehabilitation. Today, their scientific successors are deploying approaches like artificial intelligence and robotics to delve deeper into the workings of the nervous system and to restore abilities lost through injury or disease, such as creating prosthetic arms that can feel.
Can You Hear Me Now?
Clark and Hochmair-Desoyer did not invent cochlear implants, but they and their colleagues dramatically improved the technology. Clark, whose father was partially deaf, gave up a career as an ear, nose, and throat surgeon to begin working on the devices. “I got tired of taking out tonsils,” he says. Hochmair-Desoyer was a 22-year-old student studying electrical engineering — she was the first woman in Austria to earn a PhD in the field — when a colleague asked her and her future husband, Erwin Hochmair, to design an implant.
Clark’s and Hochmair-Desoyer’s teams worked independently but had to overcome common engineering obstacles. For one thing, they had to work out how to process speech — how to turn it into electrical impulses that would provide the right stimulation to the cochlea. They also had to find a better way to deliver that stimulation. The experimental implants of the time provided only a single channel of output, sending electrical impulses to only one section of the cochlea. However, different parts of the structure are sensitive to different sound frequencies. The initial designs from the Australian and Austrian researchers were multichannel devices that used several electrodes to feed impulses to the appropriate portions of the cochlea.
One of Hochmair-Desoyer’s colleagues implanted the first multichannel cochlear implants into two patients in late 1977 and early 1978, just a few months before Rod Saunders, Clark’s patient, received his. Multichannel devices represented a milestone because they were “not just a lip-reading aid,” says Clark. What recipients heard “was almost like normal speech. You could have a normal conversation,” he says.
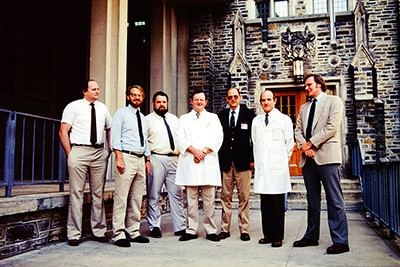
Blake Wilson (far right) and colleagues at the Baker House within the Duke Hospital during the 1980s; the team created a new speech processing procedure that transformed cochlear implants.
Image courtesy of Blake Wilson
Still, by the late 1980s only about 10,000 people in the world had received cochlear implants. Despite the technological advances, many recipients found speech unintelligible. Wilson, an electrical engineer, and his colleagues realized that the devices’ speech-processing strategy was a major reason. One problem was that electrodes in different parts of the cochlea fired at the same time and could interfere with each other, making speech sound murky.
The new strategy that Wilson and colleagues introduced in the early 1990s, known as continuous interleaved sampling (CIS), refined speech processing in several ways. In their approach, electrodes in different parts of the cochlea fired slightly out of sync, thus reducing interference. This new strategy “helped make muddy and usually incomprehensible sound far clearer than before,” says Wilson. He and his colleagues found that it increased speech comprehension by almost 50%, on average. As a result, the popularity of cochlear implants surged. CIS remains in widespread use. Moreover, most of the speech-processing approaches developed since are based on CIS.
A Stimulating Alternative to Surgery
Cochlear implants resurrect hearing by sending electrical stimulation directly to part of the nervous system. Work by the winners of a 2014 Lasker Award, Mahlon DeLong of Emory University and Alim Louis Benabid of Joseph Fourier University in France, revealed how electrical stimulation of a different portion of the nervous system could relieve symptoms of Parkinson’s disease. The treatment the researchers helped develop, known as deep-brain stimulation (DBS), uses electrodes implanted in the interior of the brain to deliver electrical impulses to certain structures. “It’s clear that DBS has greatly improved quality of life in patients with Parkinson’s disease and other movement disorders,” DeLong says.
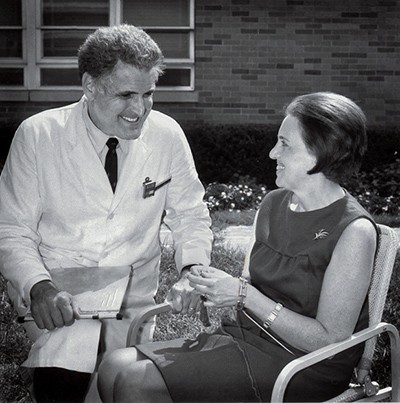
George Cotzias chats with a patient who was able to resume knitting after treatment with L-DOPA.
Image courtesy of Brookhaven National Laboratory
The symptoms of Parkinson’s disease result in part from the demise of neurons in a region of the brain called the substantia nigra, which belongs to a network of structures that help orchestrate movement. Cells in the substantia nigra release a neurotransmitter called dopamine that helps govern the activity of neurons in this circuit. As dopamine levels wane, patients develop symptoms such as tremor, rigid muscles, and difficulty moving.
In the 1960s, neuropharmacologist George Cotzias of the Brookhaven National Laboratory in New York made a treatment breakthrough. Other researchers had tried to boost dopamine levels in patients with Parkinson’s disease by administering small doses of the compound L-DOPA, which the body transforms into dopamine, but this approach produced little benefit. Cotzias and colleagues found that higher doses over longer periods eliminated tremors and restored movement in many people. He received a Lasker Award in 1969 for the discovery. L-DOPA, which one expert called “one of the most potent therapies in all of neurology — indeed, in all of medicine,” remains the centerpiece of Parkinson’s disease treatment. Its effects often dwindle, however, and it can provoke abnormal movements.
DeLong’s research set the stage for a different treatment. In the 1960s, he began probing the circuit that includes the substantia nigra, deciphering how interactions among its components enable specific movements. His findings and those of other researchers implicated one of the circuit’s structures, the subthalamic nucleus, in Parkinson’s disease. By studying monkeys with a similar illness, DeLong discovered that cells in the subthalamic nucleus become hyperactive and spur another structure in the circuit that inhibits movement to also become hyperactive, leaving patients less mobile.
In 1990, he and his colleagues revealed that they could partially reverse the process. They started by dosing monkeys with a drug that damages the substantia nigra and sparks Parkinson’s disease symptoms. Then the scientists injected the animals with a different molecule that attacks and inactivates the subthalamic nucleus. Within minutes, the monkeys started moving their limbs, and soon they were grooming themselves. “The benefit was rapid and dramatic,” says DeLong. The results raised the possibility of targeting the subthalamic nucleus to alleviate Parkinson’s disease symptoms. Disabling the structure was not an option for patients, however, because it can cause uncontrollable limb movements.
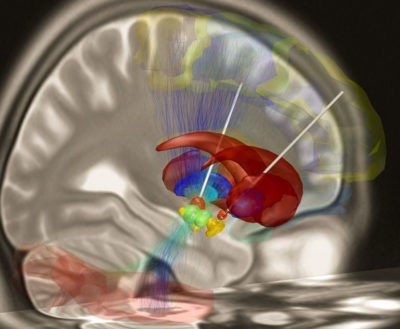
Deep-brain stimulation electrode placement
Benabid and his team found an alternative. To curb tremors, patients with Parkinson’s disease or other illnesses sometimes underwent surgery to destroy a small amount of tissue in the brain structure called the thalamus, which communicates with the movement control circuit. A neurosurgeon as well as a researcher, Benabid had performed these operations and knew that they did not always work and could cause permanent side effects. He and his colleagues had been experimenting with electrical stimulation to treat other neurological conditions, including tremor. In the early 1990s, they tried the approach in patients with Parkinson’s disease. When the researchers delivered a current to the thalamus, the patients’ tremors stopped, but their movement remained impaired.
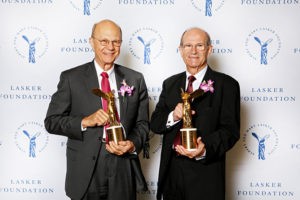
Mahlon DeLong (left) and Alim Louis Benabid with their 2014 Lasker Awards
After DeLong’s paper was published, Benabid realized that stimulating the subthalamic nucleus might be a better strategy. He and his colleagues revealed in 1998 that this approach caused patients’ tremors to subside, relaxed their rigid muscles, and improved their ability to walk and move their limbs. Since then, more than 100,000 patients with Parkinson’s disease have received DBS. Although the therapy is not a cure, it can allow patients to once again perform daily activities with fewer or no symptoms of Parkinson’s disease or the side effects of L-DOPA. In addition, DeLong notes, “the field is moving to earlier intervention in the course of the illness and to widespread use for myriad other neurologic and psychiatric disorders.”
Dissecting the Synapse
Researchers would not have been able to develop cortical implants or deep-brain stimulation without fundamental knowledge about the brain’s organization and function. Lasker winners, including recipients of a 1983 and a 2013 Award, have provided some of this knowledge. Brain neurons communicate with each other at junctions called synapses. One of the 1983 Lasker Laureates, Eric Kandel of Columbia University, discovered that certain molecular changes to synapses forge short-term memories, whereas different alterations create long-term memories. His work also earned him a Nobel Prize in 2000.
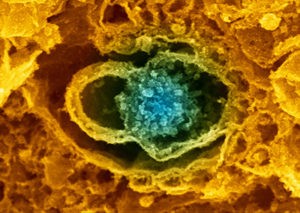
Synapse showing neurotransmitter vesicles
Image courtesy of Wellcome Collection
The 2013 winners, Thomas Südhof of Stanford University and Richard Scheller of Genentech, independently identified several proteins that permit cells to transmit signals across synapses and then teased out the functions of these molecules. Neurons talk to each other wirelessly — they do not touch at the synapse but are separated by a small space. To communicate with its neighbor, a neuron releases molecules called neurotransmitters that cross this gap. Until the neurotransmitters are needed, they remain in containers called synaptic vesicles. When a neuron is ready to dispatch a message, the vesicles merge with the cell’s membrane, liberating the neurotransmitters.
Just about everything we do, from hitting a tennis ball to enjoying a Beethoven symphony, requires the release of neurotransmitters. When Südhof and Scheller began their studies in the 1980s, however, researchers knew little about how neurons choreograph the process. Over the next two decades, the scientists filled in many of the molecular details. For instance, Scheller and his colleagues uncovered a key protein fastener on vesicles and demonstrated that it interacts with other proteins to form a molecular coupler, which helps hold vesicles in position at the cell membrane. Südhof and his team discovered a protein that prevents vesicles from prematurely merging with the membrane and another that senses calcium, the trigger for neurotransmitter release. Südhof shared a Nobel Prize in 2013.
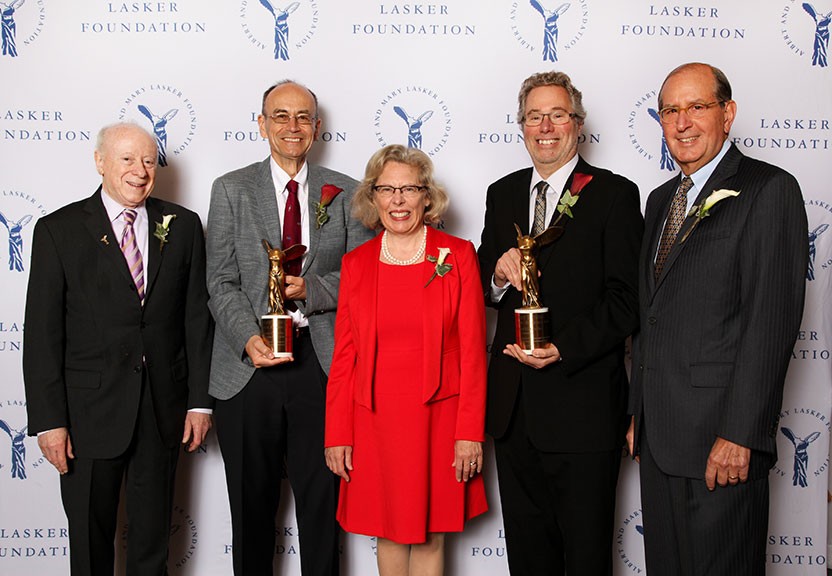
Left to right: Joe Goldstein (Jury Chair), Thomas Südhof, Claire Pomeroy (Lasker President), Richard Scheller, and Al Sommer (Board Chair) at the 2013 Lasker Awards ceremony
Capitalizing on AI and Robotics
Today, researchers are extending the work of these Lasker winners and other scientists to deepen our knowledge of the brain and provide new options for patients. For example, scientists are applying powerful artificial intelligence approaches to probe cognition and model how we think and perceive the world, says neuroscientist Nikolaus Kriegeskorte of Columbia University. “We are in a period of rapid progress for brain science.”
This work relies on complex computer models called deep neural networks, which can learn to execute a certain task, such as identifying the subjects of photographs. These networks have proven valuable, particularly for understanding vision. “They enable researchers to test hypotheses about how the brain achieves the complex computations required for perception and cognition,” Kriegeskorte says. Cochlear implants were revolutionary because they were the first prostheses to restore an impaired sense. But reviving hearing is just the beginning of what researchers hope to accomplish in rehabilitation by deploying artificial intelligence, robotics, and other approaches. Scientists are developing a variety of prostheses that receive commands from the brain and allow patients to control them. If someone has lost part of an arm in an accident, for instance, an electronic device implanted in the remainder of the limb could read nerve signals from the brain that orchestrate movement and use them to operate a prosthetic arm. Researchers have also created brain implants that allow paralyzed patients to employ their thoughts to move a prosthetic arm or even one of their own immobile limbs.
Like cochlear implants, these prostheses only transmit impulses in one direction — they do not provide sensory feedback to the brain. Biomedical engineer Daniel Ferris of the University of Florida says that researchers are now working to “close the loop” and create prostheses that have a sense of touch. That ability is crucial, he says, because “if you don’t feel what your leg or arm is doing, it’s hard to control.”
Several labs are already testing experimental prosthetic limbs outfitted with a sense of touch. The limb integrates so well with the nervous system that it “becomes part of the participant’s body image,” says Gregory Clark, who is studying one prosthetic arm model. Subjects are reluctant to give up the arm when they go home, he says. Commercial models with a sense of touch could be available in as little as five years, he adds.
Implanting devices to capture brain signals requires surgery, but researchers are also exploring if they can eavesdrop on the brain through EEGs, which record the organ’s electrical activity. For an EEG, patients only need to don a cap outfitted with electrodes, making the approach much less invasive, notes neurotechnologist Damien Coyle of Ulster University, UK. Early experiments have shown that by analyzing EEG patterns and transforming them into nerve control signals, researchers can provide paralyzed patients with some control over their limbs, enabling them to walk a short distance with assistance from a walker and harness. Scientists are testing other applications for this type of mindreading brain-computer interface, such as potentially allowing people who have suffered strokes or brain damage to communicate and receive improved stroke rehabilitation therapies.
Whether any of these new technologies will have the impact of cochlear impacts or deep-brain stimulation remains to be seen. But Graeme Clark says he is optimistic that biomedical engineers will be able to deliver advances that improve our health. “I think we are on the cusp of an exciting new era,” he says.
By Mitchell Leslie