In 1958, David Weatherall was a newly qualified doctor eager to set up a medical practice. But first he had to complete two years of national service, then mandatory in the United Kingdom. “I applied to stay in the UK because I didn’t like flying or snakes or fighting,” he recalled. Instead, he ended up serving where he might have to face all three fears—the tropical country then known as Malaya, which was combating communist rebels. Even though Weatherall’s expertise was adult medicine, the British Army put him in charge of the pediatric ward at the military hospital in Singapore.
That “series of characteristically bizarre decisions by the British Army,” as Weatherall described it, set the course for his career. One patient he saw at the hospital, the 5-year-old daughter of a soldier from Nepal, had such severe anemia that she needed blood transfusions every six weeks. Her case had mystified other doctors. But Weatherall and a colleague determined that the girl suffered from thalassemia, an inherited blood disorder that doctors then thought occurred mostly in the Mediterranean region. Weatherall stayed in the army only two years, but the experience “switched me on,” he said, persuading him to pursue research on disorders such as thalassemia that involve hemoglobin defects.
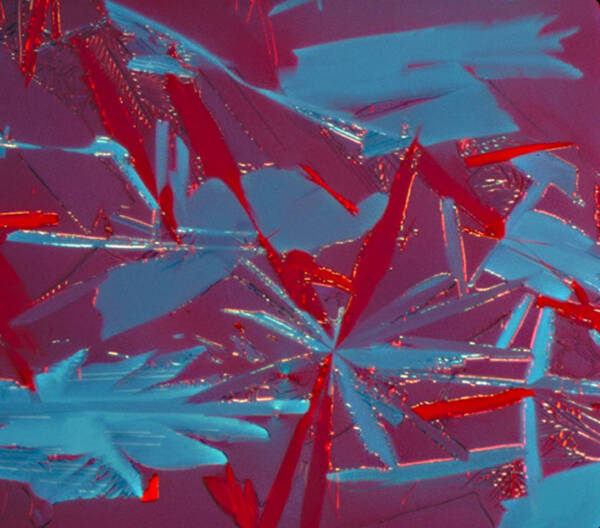
Light micrograph of hemoglobin crystals
Courtesy of Dennis Kunkel Microscopy-Science Source
Weatherall later made key discoveries about how hemoglobin abnormalities lead to the illness, which today affects 280 million people in much of the world. He also profoundly affected thalassemia research by organizing the complex and sometimes confusing findings about the disease, says David Nathan, a pediatric hematologist at Boston Children’s Hospital. “He straightened out the field.” In addition, Weatherall, then at the University of Oxford, helped launch one of the world’s first institutes for turning molecular biology discoveries into treatments. For his achievements, he earned the 2010 Lasker~Koshland Award for Special Achievement in Medical Science.
Weatherall is one of several Lasker Award winners whose work helped illuminate, treat, or diagnose genetic diseases. Those researchers brought genetics to the forefront of medicine, forged an important new way to study inherited illnesses, pioneered the use of DNA for diagnosis, and helped uncover faulty genes behind breast cancer and Huntington’s disease.
Hemoglobin’s Ins and Outs
Some of Weatherall’s most important studies came during two stints at Johns Hopkins University in the 1960s. When he left the army, researchers knew that thalassemia had two main types, alpha and beta, but didn’t understand why the forms differed. A hemoglobin molecule contains two types of amino acid chains, also known as alpha and beta. Teaming up with two other scientists, Weatherall used a new technique to measure the amount of each type of hemoglobin chain in patients’ immature blood cells. As the researchers revealed in 1965 in Nature, patients with alpha-thalassemia cranked out fewer alpha chains than normal, whereas patients with beta-thalassemia made fewer beta chains.
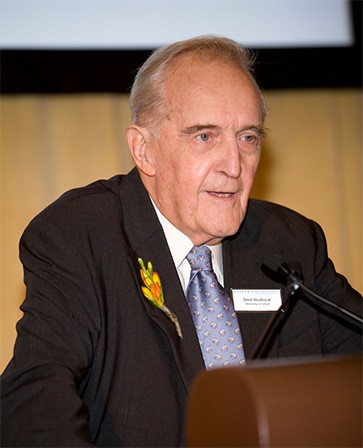
Weatherall presents his remarks at the 2010 Lasker Awards Ceremony.
In 1974, after he had returned to the UK, Weatherall and colleagues discovered why that shortfall occurs in certain patients. The researchers determined that some newborns with a fatal form of alpha-thalassemia were missing all four genes for the alpha chain. Yuet Wai Kan, winner of the 1991 Albert Lasker Clinical Medical Research Award, and colleagues independently made the same discovery. The two teams showed for the first time that losing specific genes could lead to a disease.
Weatherall also helped boost basic knowledge about thalassemia. He traveled the world, identifying and describing new disease variants. And he wrote the standard reference in the field, which compiled the expanding information about the illness. The book was “the product of a brilliant mind that could encompass this rapidly growing literature,” Nathan says.
To help patients benefit from discoveries in molecular biology, in 1989 Weatherall helped found a new facility, now known as the MRC Weatherall Institute of Molecular Medicine, where researchers and doctors can collaborate to develop new therapies.
“Weatherall was a giant in the field,”
Nathan says.
Passing the Test
Like Weatherall, Kan became fascinated with thalassemia after treating a young patient with the disease. Soon after, Kan moved to Boston to perform postdoctoral research with Nathan, who wanted to develop ways to diagnose thalassemia and sickle cell anemia—another inherited blood disorder that results from flawed hemoglobin—in the womb. In 1972, the two reported a protein-based method for prenatally diagnosing sickle cell anemia, but the procedure was cumbersome because it required fetal blood, Nathan recalls.
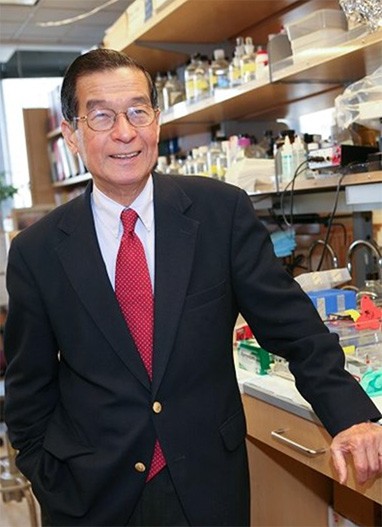
Kan in his Laboratory at UCSF
After Kan took a faculty job at the University of California, San Francisco, “he streaked ahead in prenatal diagnosis,” Nathan says. Kan and colleagues capitalized on the discovery of an enzyme called reverse transcriptase, which makes a DNA copy of an RNA molecule. Researchers can use the copy to test DNA samples for the presence of a specific gene. Kan and collaborators first applied the approach in 1974 to patients with severe alpha-thalassemia to measure how many copies of the gene for the hemoglobin alpha chain they carried—the study that mirrored Weatherall’s findings.
The researchers realized that the method had practical promise. “We immediately translated this finding to a clinical test as we soon encountered a patient who had had a previous fetus with this lethal condition,” Kan wrote. By testing cells from the patient’s amniotic fluid, in 1976 Kan and his group determined that her fetus had alpha-thalassemia. The finding was a major advance because it was the first time that anyone had used DNA to diagnose a disease.
Kan’s team also harnessed restriction enzymes: proteins that slice DNA molecules at particular nucleotide sequences. When researchers cut a piece of DNA with a specific restriction enzyme and then separate the fragments, a distinctive pattern results. Kan and colleagues found that those patterns allowed diagnosis of sickle cell anemia before birth, and the approach led to tests for other genetic diseases, including retinitis pigmentosa and cystic fibrosis. Ultimately, the discovery also offered a new way for researchers to map the location of genes. The sites where enzymes make their cuts can serve as landmarks, or markers. Mapping with restriction enzymes proved vital for tracking down many disease-causing genes, including the gene for Huntington’s disease, and helped researchers decipher the human genome. Uncovering the potential of those enzymes was “an enormous achievement,” Nathan says.
Knockout Mice Are a Hit
Knockout mice, in which a particular gene is disabled or “knocked out,” have helped researchers probe important questions, such as how arteries clog, how lung damage occurs in cystic fibrosis, and how Alzheimer’s disease disrupts the brain. Over the last 35 years, scientists have generated thousands of kinds of knockout mice, thanks mainly to the winners of the 2001 Albert Lasker Basic Medical Research Award: Mario Capecchi of the University of Utah, Oliver Smithies of the University of North Carolina, and Martin Evans of the University of Cardiff. The trio developed a method known as gene targeting for modifying specific locations in the genome, an accomplishment for which they also shared the 2007 Nobel Prize in physiology or medicine.
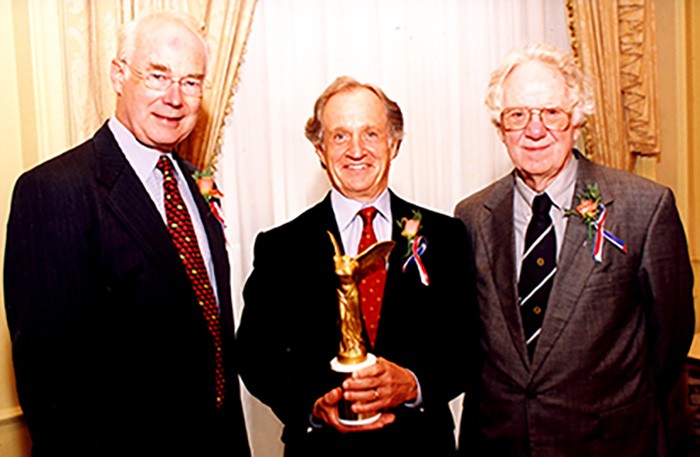
Evans (left), Capecchi (middle), and Smithies (right) at the 2001 Lasker Awards Ceremony
Working independently, Capecchi and Smithies were seeking a way to insert DNA into specific locations in the genome. Both researchers realized that homologous recombination—a natural process for fixing broken chromosomes—might allow them to direct a piece of DNA to the right spot. Homologous recombination works because our chromosomes come in pairs. To fix damage to one chromosome, cells weave in DNA from its counterpart and then fill the remaining gaps. As Smithies explained, the researchers wanted to “trick” homologous recombination into using a piece of DNA they had added.
Other scientists were skeptical. Capecchi recalled that he had submitted a grant proposal in 1980 for funding to pursue the research. The experts who reviewed the application told him not to bother. Nonetheless, over the next several years the two groups of researchers devised methods that allowed them to target genes. Smithies’s and Capecchi’s labs reported their results within a few months of each other in late 1985 and early 1986.
Scientists now knew that gene targeting “was feasible,” Capecchi wrote. It wasn’t practical, however. Only one in 1 million cells in Smithies’s experiments incorporated the DNA at the right location. To produce mice with altered DNA, the researchers would need to improve the odds of success. Evans had a solution. His lab had discovered that early embryos harbor embryonic stem (ES) cells that can differentiate into all types of body cells. In 1986, Evans and colleagues used a virus to ferry a DNA sequence into ES cells. They injected the cells into early embryos, which were then implanted into female mice. The surrogate mothers gave birth to pups that carried the viral DNA. In turn, the offspring transmitted the DNA to their young.
Capecchi and Smithies independently realized that ES cells would allow them to incorporate their gene modifications into mice. Within three weeks of each other, they contacted Evans to request some of his cells. In 1987, the two groups showed that their techniques could change a specific gene in the cells. Two years later, the first knockout mice made their debut. Today, researchers can make knockout mice with other approaches, such as CRISPR, but gene targeting with homologous recombination remains a standard technique. And scientists continue to study genetic diseases with the animals.
Hunting Down Huntington’s Disease
Nancy Wexler had never heard of Huntington’s disease when her mother was diagnosed with the inherited neurological illness in 1968. Scientists and doctors weren’t much better informed. They didn’t know what caused the disease and had no idea how to treat it. Today, researchers have nabbed the faulty gene responsible for the disease and have a much better understanding of how the genetic defect produces that fatal illness. And treatments that slow or halt its progression could be imminent. More than a dozen clinical trials to test potential therapies are running now or are in the works. Wexler, the winner of the 1993 Albert Lasker Public Service Award, was instrumental in those advances, researchers say. “Nancy deserves the credit for spearheading the effort,” says Joseph Jankovic, a neurologist at Baylor College of Medicine.
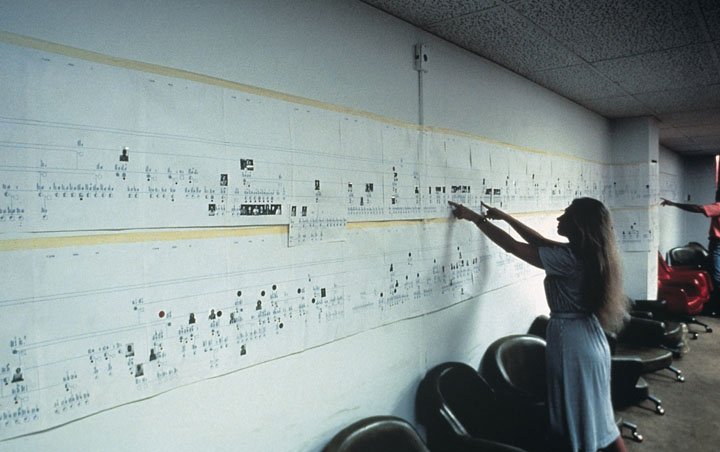
Wexler and her colleagues compiled a massive pedigree of Venezuelan families in which Huntington’s disease was prevalent.
Courtesy of Steve Uzzell, HDF
“She had initially been told, ‘Leave it to the experts,’” says neurologist Claudia Testa of the University of North Carolina School of Medicine. “Instead of sitting back, she did what had to be done.” That work included serving as executive director of the Commission for the Control of Huntington’s Disease and Its Consequences, which the US Congress set up in 1976 to identify ways to improve treatments and patient care. Wexler, now a neuropsychologist at Columbia University, also threw herself into the work of the Hereditary Disease Foundation, which her father started to secure funding for Huntington’s disease research.
Wexler’s efforts sparked important discoveries about the disease. In the late 1970s, for example, she and colleagues began studying communities near Lake Maracaibo in northern Venezuela, where Huntington’s disease was unusually common. Over more than 20 years, the team gathered samples of blood and other tissues from residents and created a gigantic pedigree chart that included more than 18,000 people from area families. “Without her leadership and inspiration, I don’t think it could have been done,” Jankovic says. That wealth of data proved indispensable, allowing scientists in 1983 to narrow the location of the Huntington’s gene to one arm of chromosome 4. That was the first time that researchers had pinpointed the chromosomal home of a disease-causing gene. Ten years later, another group of scientists identified the gene.
One reason Wexler succeeded is her gift for recruiting top scientists, says neurologist Stefan Pulst of the University of Utah. “She is an enthusiastic, smart person who could convince others to jump on the bandwagon and identify the gene. I would love to know what her secret sauce is for that power.” Equally important, she coaxed scientists, who are competitive and often reluctant to share data, to join forces. “She created an environment where people got along and worked together as much as possible,” says Albert La Spada, a neurogeneticist at the University of California, Irvine.
The discovery of the Huntington’s gene has not yet yielded a therapy that can alter the disease’s course. But Wexler, who announced in 2020 that she had inherited the faulty gene and developed the disease, remains positive. “I am optimistic that we will develop a treatment soon,” she says. La Spada agrees. “We have a number of very promising leads,” he says. “It’s inconceivable that by the end of the decade we won’t have a significant disease-modifying therapy.”
Finding a Cancer Culprit
BRCA1 is notorious. Inheriting a faulty version of the gene dramatically increases the odds of developing breast cancer and boosts the risk of ovarian, pancreatic, and prostate cancers. The winner of the 2014 Lasker~Koshland Award for Special Achievement in Medical Science, geneticist Mary-Claire King of the University of Washington, identified BRCA1. The discovery “is of monumental importance,” says cell and molecular biologist Roger Greenberg of the University of Pennsylvania. It helped elucidate the mechanism of breast cancer, paved the way for new treatments, and permitted genetic testing so that women can learn their BRCA1 status.
“Women who learn they carry a damaging mutation in BRCA1 can take action to prevent breast and ovarian cancer, and go on about their lives without the sword hanging over them,”
says King.
When King began investigating the role of genetics in breast cancer in the mid-1970s, researchers knew that the disease often ran in families. However, whether breast cancer stemmed from a single defective gene—or even whether it was inherited and not due to environmental effects—wasn’t clear. The data that King needed to resolve the issue came from a survey, conducted by the National Cancer Institute (NCI), to gauge the risk of breast cancer in women using birth control. “I asked if I could add some questions to the NCI questionnaire about family history, and the investigators very kindly said yes,” King recalled.
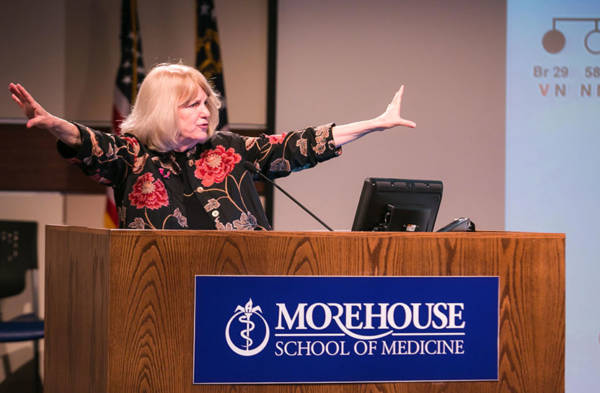
King speaking about biomedical research to students at Morehouse School of Medicine (2016)
Image courtesy of Morehouse School of Medicine
From the responses, she and colleagues gleaned more than 1,500 families in which someone had been diagnosed with breast cancer before the age of 55. When King and her team statistically analyzed the inheritance of breast cancer in that group, the results suggested that a single gene was responsible in some families. The chances that a woman with the faulty version of the gene would develop breast cancer were more than 80%, the researchers revealed in 1988, versus about 8% for women who didn’t have the mutated gene. That analysis yielded some of the first evidence that a single gene “conveyed overwhelming risk” for developing the disease, says Aravinda Chakravarti, a human geneticist at the NYU Grossman School of Medicine. “It was astonishing.”
Now King and collaborators needed to track down the gene. They started out by studying blood samples from 23 families in which breast cancer was prevalent. The researchers determined whether patients who developed breast cancer carried particular chromosome markers. If so, those markers might sit near the breast cancer susceptibility gene. Their findings, reported in 1990, suggested that the gene, which they named BRCA1, was on chromosome 17. King said she was a little unsure about the results until another lab confirmed them. “Then we had champagne.” That discovery set off a race among about 15 scientific teams, including King’s, to sequence BRCA1. Researchers from the biotech company Myriad Genetics published their results first, in 1994. “I had thought I would be devastated if we didn’t ‘win the race,’ but I wasn’t,” says King. “It was a tremendous relief to have the gene to work with, to design diagnosis and treatment.”
Identifying BRCA1 made a big difference for patients. Now, women with a family history of breast or ovarian cancer can undergo genetic testing to determine whether they carry the mutated version of the gene. If so, they may choose to have surgery to remove their breasts or ovaries, which “reduces risk by about 90%,” Greenberg says. And if patients develop cancer, their BRCA1 status shapes their treatment. Drugs known as PARP inhibitors are available to combat cancers with mutations in BRCA1 or its cousin, BRCA2.
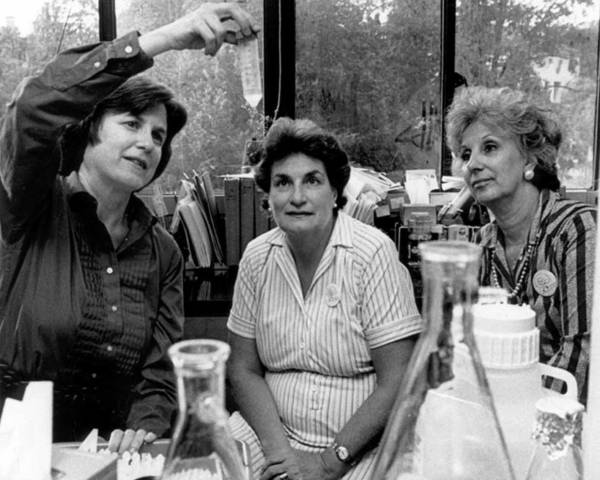
King (left) talks with Abuelas de Plaza de Mayo, Estella Carlotto (right) and Nelida Gomez (center), about developing genetic tests to reunite families with grandchildren who were kidnapped during the “Dirty Wars” in Argentina.
Image courtesy of The National Academies of Sciences, Engineering, and Medicine
King’s Lasker Award also honors her work to apply DNA technology to identify people killed or kidnapped during conflicts. For example, in 1983 King agreed to assist a group called Grandmothers of the Plaza de Mayo in Argentina. The women were trying to find their lost grandchildren, who had been kidnapped by the military dictatorship that ruled the country in the 1970s and early 1980s and put up for adoption. Thanks to King and colleagues, 82 children reunited with their grandparents. She also has helped relatives identify victims of political violence in other parts of the world, including Rwanda, Bosnia, and El Salvador.
Taking Genetics out of the Lab
In the late 1950s, Victor McKusick chose to commit professional suicide—or at least that’s what many colleagues told him. A respected cardiologist at Johns Hopkins University and an authority on diagnosing cardiovascular diseases by listening to the heart, McKusick decided to switch to genetics. At the time “most physicians ignored it because they were looking for communicable factors like microbes” as the causes of disease, Chakravarti says. But McKusick saw the value of the fast-expanding knowledge about inheritance and disease, and he ended up launching a new field, medical genetics. “Victor conceptualized the field” and his work showed “what genetic medicine could be,” Chakravarti says.
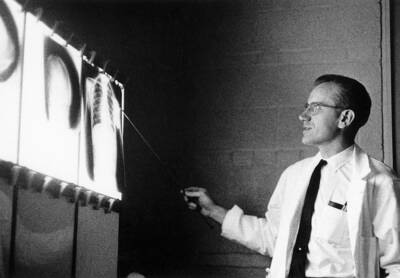
Victor McKusick points to a feature on an x-ray while lecturing at Johns Hopkins University in the 1950s.
Courtesy of The Alan Mason Chesney Medical Archives
One way in which he helped establish the discipline was by launching the world’s first medical genetics clinic. It not only treated patients but also trained doctors and other clinicians and performed research. As part of their studies, McKusick and colleagues sought to document as many genetic diseases as possible. Their research on the Old Order Amish in the United States revealed several new genetic conditions, for instance. “He wrote incessantly about this subject,” Chakravarti says. His most important work was a catalog of all known genetic diseases, Mendelian Inheritance in Man, published in 1966 and which continues today as an online encyclopedia.
Genetics is now an essential part of medicine. Genetic testing for diagnosis is routine, for example, and researchers have devised therapies for some inherited disorders. But McKusick had a broader impact. In 1969 he proposed a project to map the chromosomal locations of all human genes. When researchers finished sequencing the human genome more than 30 years later, Francis Collins, then head of the National Human Genome Research Institute, told an interviewer, “Victor McKusick started this whole thing in motion. He’s the guy who led us here.”
McKusick and the other Lasker winners who studied genetic diseases left an important legacy. Their work has paid off for the patients who have benefited from new diagnostic tests and targeted treatments. And more such advances are in store as researchers continue to build on their discoveries.
By Mitchell Leslie