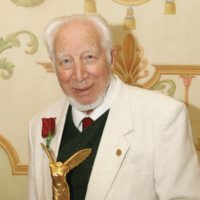
Willem J. Kolff
University of Utah School of Medicine
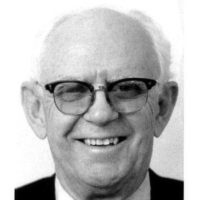
Belding H. Scribner
University of Washighton
For the development of renal hemodialysis, which changed kidney failure from a fatal to a treatable disease, prolonging the useful lives of millions of patients.
This year’s Lasker Clinical Medical Research Award honors two scientists who changed kidney failure from a fatal to a treatable disease. By developing the artificial kidney and devising a system for repeating hemodialysis over a period of months and even years, Willem Kolff and Belding Scribner, respectively, have prolonged the useful lives of millions of people.
The fate of kidney patients has undergone a revolution in the last half century, due in large part to Kolff’s and Scribner’s seminal contributions. The kidney filters metabolic byproducts from the blood, and when it fails, patients suffer from a variety of symptoms, including weight loss, nausea and vomiting, gastrointestinal hemorrhaging, itching, lethargy, convulsions, and coma. Without treatment, death ensues. Hemodialyzers replace the cleansing capabilities of the kidney, and although the organ performs other physiological tasks as well, the machine’s ability to extract impurities bestows vitality upon formerly doomed individuals.
In the late 1930s, Kolff decided to devise a contraption that would clean up tainted blood from acutely ill patients, thereby ushering them through brief periods of time in which their kidneys failed to function. Today, a variety of problems can temporarily disable a kidney, such as complications from antibiotic treatment, extensive muscle injury due to trauma, or autoimmune diseases such as systemic lupus erythematosus. Under adverse conditions – the German’s had invaded the Netherlands, where Kolff lived and worked – he plugged away, and by the mid-1940s, he had succeeded. He developed the first clinically useful hemodialyzer, a machine that removes toxins by encouraging them to migrate from a patient’s blood into a rinsing bath before returning the blood to the patient. With this treatment, the death rate from acute kidney failure plummeted.
Kolff’s breakthrough was the first of two major advances that brought dramatic new hope to kidney patients. Fifteen years later, Scribner imagined an apparatus that would extend the benefits of Kolff’s invention to patients with chronic kidney disease. Medical conditions such as diabetes or high blood pressure can permanently incapacitate the organ, producing so-called end-stage renal disease (ESRD). In Kolff’s procedure, surgeons inserted needles to access arteries and veins that sent the blood to the hemodialyzer and then received it back into the body. After a limited number of times, such operations battered the circulatory vessels so badly that they could not be reused. Scribner concocted a U-shaped device, or shunt, that plugged into one artery and one vein – and could stay there indefinitely. Medical staff would hook it up to the machine during dialysis and then re-cap it to restore normal blood flow through the body.
These innovations provided life support for individuals with kidney failure. Today, hemodialyzers remain the only external life-sustaining machines that replace a failed organ. The Kolff and Scribner instruments – and others derived from them – have profoundly transformed patients’ chances of surviving a range of kidney malfunctions. Before Kolff’s invention, mortality from acute renal failure was about 75-90 percent; now it has dropped to about 20-50 percent, depending on the nature of the underlying disease. The Scribner shunt has dramatically expanded the utility of the hemodialyzer, bringing it to ESRD patients. More than 350,000 patients in the United States are living with ESRD, and more than 250,000 of them are on chronic dialysis. The others, transplant recipients, flow in and out of the dialysis pool. Worldwide, chronic hemodialysis helps or has helped more than 1.5 million individuals.
The ability to filter impurities from patients’ blood over long periods of time has spurred research into other aspects of kidney function that must be restored in order to faithfully mimic the natural organ’s activities. Moreover, it has permitted the kidney transplantation field to blossom, which has benefited more than 100,000 people in the U. S. alone and sparked many fundamental and applied advances in the field of immunology.
Hemodialysis has become a universal standard of care for individuals with acute and chronic kidney failure. Furthermore, it is the first – and still the only – method in which a machine replaces a failed internal organ. Kolff’s and Scribner’s contributions have touched millions of people across the globe, and have reached far beyond the field of kidney biology.
Life-saving Cellophane – More Than a Sausage Casing
In 1938, recent medical school graduate Willem Kolff faced a frustrating and painful situation. At the University of Groningen hospital in the Netherlands, he cared for a young man who was suffering from kidney disease. Kolff watched helplessly as toxic waste buildup slowly shut down his patient’s body and eventually claimed his life.
If only Kolff could find a way to extract the poisons produced each day, he reasoned, he might be able to save such patients. While researching the subject, he found that others had shared his vision.
In 1913, the father of U. S. pharmacology John Abel of Johns Hopkins University, and his colleagues, had experimented with the concept of an artificial kidney, and had attained some success. They laced dog blood with aspirin, and funneled it through a series of tubes made of a porous material. During its journey, it came in contact – through the molecule-sized pores – with rinsing fluid that chemically resembled healthy blood, The technique drew out the aspirin, but it was not suitable for human use: It operated inefficiently and employed an unsafe substance from leech heads to prevent clotting.
In 1938, Dr. Kolff had a new anti-coagulent at his disposal: heparin. He still needed a filtration material, however. He settled on sausage casing, which was composed of a thin membrane called cellophane. Others researchers had shown that if two solutions of different chemical concentrations were separated by cellophane, molecules moved from the pool of greater concentration to that of lower concentration. In principle, Kolff could harness this phenomenon for hemodialysis: The toxins would travel from the blood inside the cellophane tube to the rinsing fluid on the other side. To derive the most benefit from this system, he would design a device with the largest possible amount of cellophane surface area to maximize the interface through which the impurities could flow.
During the next several years, Kolff built four different artificial kidneys, none of which was reliable enough for clinical use. In the meantime, war had broken out in Europe. His strongest supporter, Department of Medicine chief Polak Daniels, who was Jewish, committed suicide as the Nazis invaded the Netherlands. A Dutch National Socialist took over Daniels’s post at the University of Groningen and Kolff decided to leave. He obtained a position as an internist in the city of Kampen, and persevered in developing his artificial kidney. This quest required great ingenuity and courage due to the extreme hardship resulting from the German occupation. The Nazis had rationed everything and appropriated all useful materials for their war effort. Needles were scarce and rubber tubing was so precious that Kolff used it only where he needed flexible joints; in other places, he substituted glass tubing. The first kidneys contained aluminum strips, and when Kolff could no longer obtain the metal, he replaced it with wooden slats. They worked fine, except when they warped. Fortunately, he had foreseen his need for large amounts of sausage casing, and had bought plenty of that before the Germans arrived.
He devised a fifth prototype, and persuaded the managing director of a local enamel factory, Henrick Berk, to help him construct it. In 1942, they built the first rotating drum artificial kidney. Kolff wound 100 feet of cellophane in a spiral pattern around a large horizontal drum, which revolved in an enamel tube containing a rinsing fluid. Gravity drew blood in the cellophane to the lowest point, and as the drum turned, the blood worked its way from one end of the cellophane tube to the other, releasing its impurities into the surrounding fluid as it traveled.
Most physicians had little faith in Dr. Kolff’s invention. As a result, they would only refer him patients who were comatose and close to death. Although his initial treatments didn’t produce lasting improvements, the results encouraged him. The first 15 patients died – but not from the therapy. Furthermore, Kolff could control concentrations of urea, a key metabolic breakdown product, and some patients awoke from their comas before succumbing again to their illness.
In September, 1945, Kolff achieved his first undeniable success. Ironically, his patient was a 67-year-old woman who had collaborated with the Nazis. He encountered considerable hostility from the local citizens for trying to help her, but decided to proceed, remaining true to his role as a physician and desire to develop the artificial kidney. During the treatment, she was unconscious, and, according to an editorial he wrote in 1993, “after many hours of dialysis, I bent over her and asked if she could hear me. She slowly opened her eyes and said, ‘I’m going to divorce my husband.'” Her kidneys began to produce urine, she broke off her marriage, and lived for another seven years. Kolff had achieved his first victory against kidney disease.
After the war, he sent artificial kidneys to England, Canada, and the U. S.. By making his machines available worldwide, he catalyzed the rapid development of hemodialysis as a life-support system in patients with acute kidney failure. It remains the standard treatment today. The artificial kidney has saved innumerable lives by making the mortality rate for acute, reversible kidney failure plummet.
Kolff’s triumph with the artificial kidney was only the first step in a career that brims with fresh vision and daring inventions. He sparked the establishment of the American Society for Artificial Internal Organs, an organization that has roused tremendous research activity in the field. While improving the original kidney, Kolff branched out to tackle an array of other artificial organs. He headed a team that invented the first artificial heart, showed that it worked in animals, and adapted it for human use. In 1982, this device allowed Barney Clark to live for 112 days. Kolff also designed the intra-aortic balloon pump, a gadget that reduces the heart’s workload and improves blood flow to the coronary arteries. Surgeons use this apparatus to support the heart when it fails or during particular medical procedures. Currently in his 90s, Kolff continues to develop artificial organs.
Kolff’s hemodialysis apparatus and its descendents could preserve the life of patients with temporary kidney failure until their own kidneys began to operate again. But a single patient could benefit from the regimen only a few times; surgeons inserted tubes that connected a patient’s artery and vein to the artificial kidney, and removed them after dialysis. With repetition, this procedure thrashed the blood vessels and doctors eventually ran out of places to tap into the bloodstream. This limitation rendered hemodialysis essentially useless for the tremendous numbers of people with ESRD, who have permanently ravished kidneys and would require frequent treatment.
Teflon Technique Sticks
Scribner’s invention enlarged the province of hemodialysis to include ESRD patients. As a faculty member at the University of Washington, Seattle, Scribner encountered a gravely ill patient, whom the medical team had revived with an artificial kidney. The patient responded unusually well, and within three days, he was wandering the hospital corridors. But the cure was temporary because it turned out that he had permanent kidney damage. Despite the amazing recovery, he died soon afterward.
Several weeks later, Scribner woke up in the middle of the night with an idea about how to save such patients. A U-shaped shunt – with one end inserted into an artery and one into a vein – could remain attached permanently, For each round of dialysis, medical personnel could plug tubes into this gadget and hook them up to the artificial kidney. Blood would flow from the artery to the dialysis machine and then back to the vein. After dialysis concluded, the apparatus would be capped, which would reconnect the artery and the vein. In principle, such a piece of equipment would facilitate repeated rounds of hemodialysis.
Scribner and his associate, surgeon David Dillard, contacted engineer Wayne Quinton. Quinton soon mastered the art of bending Teflon, a process similar to glass blowing. Teflon was crucial to the device because it repels virtually all substances; before a blood clot can build up, it slips off. In addition, the material doesn’t react with tissues and can be shaped. By March of 1960, the three men were ready. They implanted the first shunt into Clyde Shields, a 39-year-old machinist who was dying of incurable kidney disease. Although kidney experts doubted that long-term dialysis would succeed because the kidney performs many functions in addition to clearing the blood of toxic metabolites, Shields would live for 11 years on intermittent hemodialysis and the same therapy would sustain Scribner’s fifth patient, Tim Albers, for 36 years. Scribner had performed a monumental feat, providing continuous replacement of an organ’s vital functions in such a way that a patient could survive with a reasonable quality of life.
Suddenly the prognosis for ESRD patients flipped from 90 percent fatal to 90 percent survivable. Scribner transformed the outlook of patients whose kidneys had failed from almost certain death to a reasonably vigorous life. Furthermore, the advance opened up the field of kidney transplantation. With the Scribner shunt, potential recipients could be sustained while they awaited donor organs.
Scribner set up the first outpatient dialysis center in the world, now called the Northwest Kidney Center, but at first, it could handle only a fraction of patient applicants. Suddenly the world of medicine confronted a perplexing question: Who should live and who should die? The first bioethics committee – consisting of lay people as well as physicians – formed to select the lucky individuals. Many of today’s bioethics discussions owe their origins to the development of hemodialysis technology that could serve a large number of people in principle, but not in practice.
Scribner played a central role in creating this ethical dilemma, but he quickly found ways to resolve it – at least with regard to hemodialysis. He and his colleagues developed a small, portable device for use in the home, and included features that allowed non-medically trained personnel to operate it safely. This innovation liberated beds in the center and also helped patients incorporate the procedure easily into their normal lives.
The Legacy
Many investigators, including Kolff and Scribner, have improved their initial designs and approaches. The Scribner shunt, for example, has been replaced by other gadgets that allow permanent access to blood vessels. Kolff’s and Scribner’s inventions and ideas, however, represent the pioneering breakthroughs that led to these later developments and all subsequent techniques have relied upon their original concepts and technology.
Furthermore, the effects of Kolff’s and Scribner’s advances have reverberated beyond the area of hemodialysis. The ability to remove toxins from the blood raised the possibility of replacing all kidney functions and galvanized research efforts aimed at devising ways to therapeutically simulate the rest of this organ’s roles. Such activities include converting vitamin D to its active form, which is required to build bone; producing erythropoietin, which stimulates production of red blood cells; and generating hormones that regulate blood pressure. As a result of this research, physicians have refined their ability to therapeutically substitute for kidney functions; for example, they now administer recombinant erythropoietin to correct the anemia that almost universally accompanies chronic kidney failure. Furthermore, the flourishing field of transplantation has brokered new insights into the immune system’s ability to distinguish self from non-self, and its response to foreign tissue.
For more than half a century, hemodialysis has been saving patients whose kidneys have failed. It has already touched millions of people, and that number grows every year. The hemodialyzer continues to provide the only effective therapy for this condition, which stemmed largely from the frustration, imagination, and persistence of two exceptionally creative medical scientists.
Award presentation by Joseph Goldstein
In one of her short stories, the Danish writer Isak Dinesen poses a provocative question: “What is man, when you come to think upon him, but an ingenious machine for turning, with infinite artfulness, the red wine of Shiraz into urine?” Well, when you come to think upon it, this remarkable conversion is carried out by a real ingenious machine, the kidney — a truly remarkable organ. The kidney not only cleanses the blood of toxic products like the red wine of Shiraz, but it also regulates with extraordinary constancy the volume and composition of the body fluids that bathe all the tissues.
Claude Bernard, the great physiologist of the 19th century, pointed out that it is this constancy of the internal environment, orchestrated by the kidney, that allowed animals to achieve a free and independent life. Homer Smith, the great physiologist of the 20th century, had a more watered-down view of the kidney: “Bones can break, muscles can atrophy, glands can loaf, even the brain can go to sleep without immediate danger to survival. But should the kidneys fail — neither bone, muscle, gland, nor brain could carry on.” This is the ultimate kidney-centric view of the world.
The development of an artificial kidney that could substitute for the body’s damaged kidneys constitutes one of the monumental life-saving advances in the history of modern medicine. Prosthetic devices for non-essential body parts, such as teeth, limbs, and even hair, have been available for centuries, but the artificial kidney is the only artificial device that can replace a vital organ on a permanent basis. The 2002 Lasker Award for Clinical Research celebrates the achievements of the two scientists who made all this possible — Willem Kolff and Belding Scribner.
Our story begins in 1938 at a small medical ward at the University of Groningen Hospital in the Netherlands. The physician in charge was Willem Kolff, who had just graduated from medical school. One of his first patients was a 22-year-old man in uremic coma. The young Dr. Kolff, then only 28 years old, watched helplessly for four days as the young man died in front of his eyes. He had no treatment to offer — if only he could find a way to remove the toxic metabolic wastes that accumulated in blood when the kidney failed. During the last day of the patient’s life, Kolff went to the university library and searched the literature for ways of purifying blood. To his delight and surprise, he found an article published 25 years earlier, in 1913, by a distinguished pharmacologist at Johns Hopkins University, named John Abel, who described a procedure for dialyzing the blood of dogs and rabbits. The blood was taken from the animal, passed through a series of porous colloid tubes that were bathed in salt solution, and then put back into the animal. Clotting of the blood was prevented with an anticoagulant called hirudin that Abel extracted from thousands of leeches obtained from Parisian barbers. In 1924–28, the German scientist Georg Haas first attempted hemodialysis on several humans with acute renal failure, but the duration of the procedure (30–60 minutes) was too short for any significant therapeutic effect.
Stimulated by Abel’s concept of hemodialysis, Kolff was determined to develop an artificial kidney that would save the lives of uremic patients. He rapidly overcame two of the technical obstacles inherent in the Abel technique: Heparin was substituted for the highly antigenic protein hirudin, and a thin cellophane membrane was used instead of a thick colloid tube. But then, Kolff faced an even more formidable obstacle: World War II had just broken out, and the Netherlands soon fell to Nazi Germany. Kolff was assigned to work in a 90-bed hospital in a small town called Kampen. Despite the difficult circumstances of Nazi-occupied Netherlands, Kolff miraculously cajoled an enamel manufacturing company to help him obtain scarce materials in order to construct the first artificial kidney. This machine, which came to be known as the “rotating-drum hemodialyzer,” consisted of 130 feet of cellophane tubing made from sausage casing, wrapped 30 times around a horizontal drum made out of aluminum strips. As the drum rotated through a bath of salt solution contained in an enamel tank, the patient’s blood was exposed to the dialysis bath, allowing rapid and efficient removal of the toxic wastes.
Between 1943 and 1944, Kolff treated 16 patients with acute kidney failure, but success was limited. The first unambiguous success came in 1945 with the 17th patient, a 67-year-old woman in uremic coma due to acute renal failure from Gram-negative sepsis. In one of the ironies of medical history, this patient was a Nazi sympathizer who had betrayed many of her Dutch countrymen to the Germans. After 11 hours of hemodialysis, the patient regained consciousness. According to Kolff, “I bent over and asked if she could hear me. She slowly opened her eyes and said, ‘I’m going to divorce my husband.'” Her kidneys began to produce urine, she recovered fully, and true to her word she divorced her husband, and lived seven more years before dying from another disease. Kolff had now achieved the first step in the conquest of kidney failure: The revolution of the drum had started a revolution that would ultimately improve the health of millions of people.
When World War II ended, Kolff donated all five of his artificial kidneys to hospitals in London, Poland, The Hague, Montreal, and Mt. Sinai Hospital here in New York City. This extraordinary act of generosity enabled physicians throughout the world to become familiar with the new technique of dialysis. He also provided blueprints of his rotating-drum hemodialyzer to George Thorn at the Peter Bent Brigham Hospital in Boston. This led to the manufacture of the Kolff-Brigham kidney, which was an improved stainless steel version of the original. The Kolff-Brigham kidney made it possible for John Merrill to establish the first major program for hemodialysis in the United States and paved the way for the first kidney transplantation by Joe Murray in 1954. Kolff-Brigham kidneys were also used during the Korean War to dialyze American soldiers who suffered massive wounds and posttraumatic renal failure.
After inventing the artificial kidney, Kolff went on to become the world’s premier biomedical engineer — inventing the pump oxygenator for open heart surgery in 1955, the aortic balloon pump for treatment of cardiogenic shock in 1961, and the artificial heart that prolonged the life of Barney Clark for 112 days in 1982. As he approaches his 92nd birthday, Kolff refuses to rust on his laurels; he is as passionate as ever in his inventive quest for new body parts, now pursuing the artificial eye and the artificial ear.
The Kolff kidney solved the problem of acute renal failure, but what about the hundreds of thousands of patients with chronic end-stage renal disease for whom prolongation of life requires repeated dialysis three times a week forever? In the late 1950s, the conventional wisdom among kidney experts was that chronic intermittent dialysis would never be possible because of two insurmountable problems — one technical and one psychological. The technical problem was one of circulatory access: Every time a patient was hooked up to a dialysis machine veins and arteries were damaged, and after six or seven treatments, physicians would run out of places to connect the machine. The psychological problem stemmed from the widely held mystical belief that a cellophane dialyzer outside the body could never permanently replace the complex functions of a normal organ. After all, according to the experts, the kidney was a sacred organ. Above and beyond its excretory function, it produces three essential hormones — erythropoietin for forming red blood cells, renin for maintaining blood volume and blood pressure, and hydroxylated vitamin D for preventing breakdown of the bones.
In 1960, the impossible suddenly became possible. The psychological and technical barriers to chronic dialysis came crashing down through the research of Belding Scribner, a young professor of medicine at the University of Washington in Seattle. Like Kolff, Scribner was a dedicated physician whose imagination was triggered by a patient who was slowly dying of end-stage renal disease. After a sleepless night agonizing over the fate of his patient, Scribner got out of bed at 4:00 a.m., and in a sudden flash jotted down an idea about how to solve the problem of circulatory access. His idea was elegant in its simplicity: sew plastic tubes into an artery and a vein in the patient’s arm for connection to the artificial kidney. When the dialysis treatment was over, keep the access to the circulation open by hooking the two tubes together outside the patient’s body via a small U-shaped device, made of Teflon. This U-shaped Teflon device, which came to be known as the Scribner shunt, served as a permanently installed extension of the patient’s own circulatory system, shunting the blood from the tube in the artery back to the tube in the vein. Whenever the patient needed to be dialyzed again, no new incisions in the blood vessels had to be made. The shunt was simply disconnected from the tubes in the patient’s arm, and the patient was hooked up again to the machine.
One of the key factors contributing to success of the Scribner shunt was the use of the then-new material Teflon, whose nonsticky properties prevented clotting of the blood. Although it has been replaced today by improved methods of circulatory access, the Scribner shunt was the technical breakthrough that transformed the outlook of patients with end-stage renal disease from a sentence of death to a prolongation of life. The first patient to receive a Scribner shunt was dialyzed repeatedly for 11 years, and the fifth patient was dialyzed for 36 years, undergoing 5700 cycles of hemodialysis, before he died several years ago.
In 1962, Scribner established the world’s first outpatient dialysis center, now known as the Northwest Kidney Center. Almost immediately, the demand to treat patients vastly exceeded the capacity of the original six dialysis machines. To make matters worse, in 1962, insurance companies and Medicare did not cover the costs of chronic dialysis, which was $10,000 per year in 1960 dollars. Dying patients were clamoring to be dialyzed. Who goes on the machine first? Scribner suddenly found himself in one heck of a pickle. His solution to the allocation problem was novel and clever. The decision to choose who should live or die would be made, not by Scribner himself, but by an anonymous committee of citizens, appointed by the local Seattle medical society, that included five lay community leaders from various walks of life and two physicians outside of the kidney field. The creation of this bioethics committee — the first of its kind — was highly controversial at the time, but in retrospect it changed the thinking about accessibility of health care in the United States.
In another innovation, Scribner ushered in the era of home dialysis by developing a miniature portable dialysis machine with fail-safe devices that could be run by family members. Today, 40 years later, nearly 300,000 patients in the United States and 1.5 million worldwide are undergoing chronic dialysis either at home or at dialysis centers. The technology of chronic dialysis has become so sophisticated that thousands of patients with end-stage renal disease take holiday cruises to exotic places anywhere in the world. Next week, for example, the MS Rotterdam leaves New York City for a two-week “Dialysis at Sea Cruise” to Bermuda and Barcelona.
The contributions of Willem Kolff and Belding Scribner revolutionized the treatment of kidney disease, saving and prolonging the useful lives of millions of people. To paraphrase Isak Dinesen, “What is the artificial kidney, when you come to think upon it, but an ingenious machine of Kolffian cellophane and Scribnerian Teflon for turning, with infinite artfulness, death into life?”