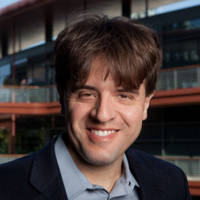
Karl Deisseroth
Stanford University
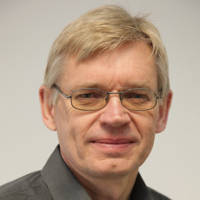
Peter Hegemann
Humboldt University of Berlin
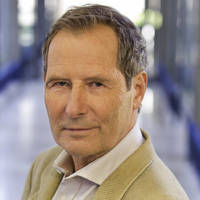
Dieter Oesterhelt
Max Planck Institute of Biochemistry
For the discovery of light-sensitive microbial proteins that can activate or silence individual brain cells and for their use in developing optogenetics—a revolutionary technique for neuroscience
The 2021 Albert Lasker Basic Medical Research Award honors three scientists for the discovery of light-sensitive microbial proteins that can activate or silence individual brain cells and for their use in developing optogenetics, a revolutionary technique for neuroscience. Dieter Oesterhelt (Emeritus, Max Planck Institute of Biochemistry, Martinsried) uncovered an archaeal protein that pumps protons out of cells when illuminated. Peter Hegemann (Humboldt University of Berlin) then found related channel proteins in single-celled algae. Karl Deisseroth (Stanford University) harnessed these molecules to create light-triggered systems that can be deployed in live, free-moving animals to decipher the role of specific classes of—and even individual—neurons within labyrinthine brain circuits. Hundreds of laboratories around the world are now using this approach, called optogenetics, to untangle the elaborate networks that underlie healthy physiology and neurological disease.
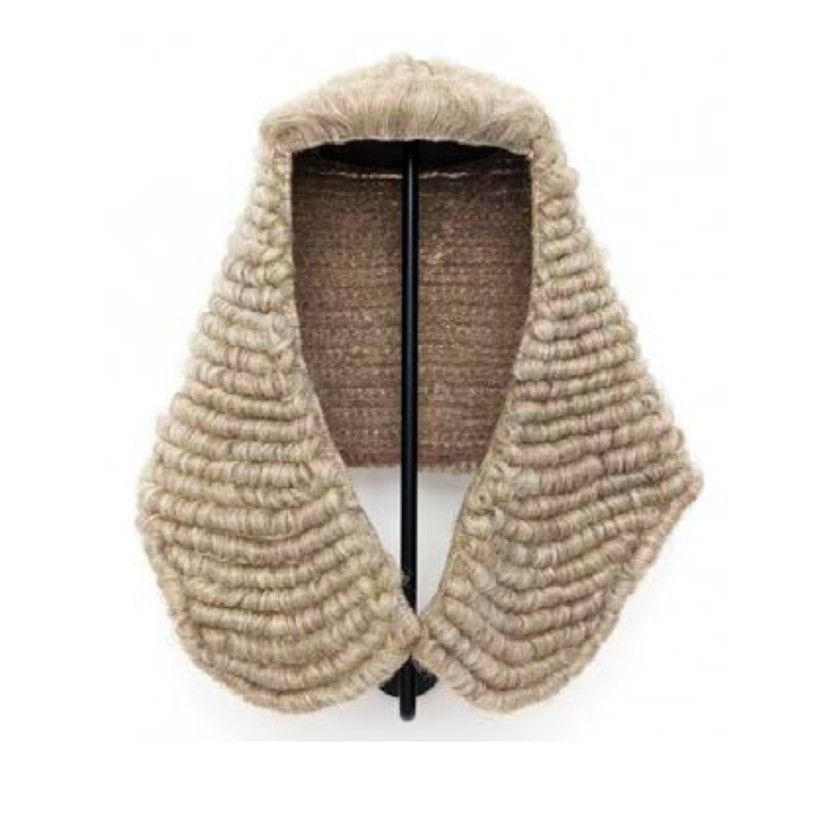
The Surprise Element: A Distinctive Characteristic of Creative Scientists, Artists, and Comedians
Truly creative works of science and art produce unexpected and surprising results—just like the punch line of a good joke that generates an unfamiliar twist on a familiar idea.
Acceptance remarks
Acceptance remarks, Karl Deisseroth
I am tremendously honored to accept this award along with my friends and colleagues Peter Hegemann and Dieter Oesterhelt. Ours has been a story of international scientific cooperation and deep friendship over many years, from our elucidation of the microbial transduction of light into ion flux, to illuminating the mysteries of emotion, in health and in psychiatric disease.
In the same sense that congestive heart failure, the weakening of a pump, is accompanied by a variety of symptoms, we must ask: What are, materially, the diverse features of debilitating psychiatric disease? Due to our limited understanding, these disorders continue to cause worldwide morbidity and mortality comparable to, or greater than, that of any other illness. While Covid-19 reshapes epidemiology, the pandemic has brought greater urgency to our search for understanding in psychiatry; the trauma and intense stress experienced across the globe have intensified symptom expression in mental illness, and most of my new patients these days frame the acute exacerbations of their suffering in terms of the pandemic and its consequences.
This hope for physical understanding did not only bring me to the field of psychiatry, but sustained me during personally difficult years of residency training, and throughout the past twenty years of caring for patients with mental illness. This aspiration also guided my laboratory to develop an approach for mapping individual features of adaptive and maladaptive brain states onto causal and precisely defined elements of neural circuitry.
My earliest formal scientific education provided insight into the capability of high-resolution mechanistic studies to reveal deep mysteries of biology. Isolating the position of individual atoms in microbial light-activated membrane proteins and elucidating their precise roles allowed us to explore the operation of biological systems as large and complex as the mammalian brain—and in so doing, to illuminate the cellular-activity basis for survival drives such as thirst and hunger, the intricate cellular dynamics involved in sensation and perception, and even complex cognitive processes such as social interaction.
Coming full circle over the past twenty years, we now can approach causal and material understanding of psychiatric disease-related states such as anxiety and dissociation, at the level of highly-resolved physical components. This story exemplifies the power of taking a molecular approach to explore complex biological systems–even for the disorders that define psychiatry. The hope brought to psychiatry by detailed studies of microbial membrane proteins is an uplifting story for all to share.
The scope of this fifty-year story also exemplifies how science proceeds in unpredictable ways: here, from a solvent-induced color change noticed by Dieter Oesterhelt while prepping lysed archaea, to the study of light responses in single-celled green algae by Peter Hegemann, to our structural and mechanistic studies together of microbial light-gated ion channels, and finally to our study of complex internal representations and behaviors by recruiting individually-specified cells with light. In these difficult times but with hope for the future grounded in science, I am deeply grateful to our lab members and collaborators over the years, and to the global community. The process of science truly brings the many faces of humanity together.
Acceptance remarks, Peter Hegemann
I am extremely grateful to receive the 2021 Lasker Award. To share the prize with my early mentor Dieter Oesterhelt and my long-standing friend and collaborator Karl Deisseroth is a special source of joy.
Dieter’s discovery of bacteriorhodopsin fifty years ago as a light driven proton pump is a milestone in photoreceptor research. For many decades bacteriorhodopsin served as a model that helped spur countless advances. It also became a template for further investigation of light-sensitive microbial proteins.
My group studied the photoreceptor molecules of Chlamydomonas and other algae over many years until we understood the function of most of the amino acids that make up the tiny biological photodiodes called channelrhodopsins. The wide repertoire of biophysical and biochemical techniques built upon the foundation of earlier research was instrumental in helping gain deeper knowledge of these remarkable proteins.
In a great collaboration with Karl, we engineered the channelrhodopsins with the hope of better understanding Nature’s concepts. Ultimately, we were able to make microbial opsins available for neuroscience applications, creating a field now called optogenetics. Karl is to be commended for transferring the knowledge about channelrhodopsins to the neuroscience community.
Progress in science to some extent depends upon risky decisions of individuals. At times the results of these decisions can only be appreciated later in one’s career. We all rely upon generous and enthusiastic coworkers and upon international collaborators who suffered and enjoyed with us the ups and downs of often painstaking work. Together, we shared both the patience and the joy that comes from working in wild unexplored territories in order to bring discoveries about tiny proteins found in bacteria and algae to unexpected applications.
I am grateful for the opportunity that this Award gives us to look back to the discovery decades ago of the first microbial rhodopsin, all the way up to the wide use of channelrhodopsin in the neurosciences, and then forward toward the promise of clinical applications of optogenetics.
I look forward to celebrating together with my co-laureates Dieter and Karl, with our coworkers and families at home, and with members of the Lasker Jury.
Acceptance remarks, Dieter Oesterhelt
Being honored with this Award brings back the times more than fifty years ago when I was a young biochemist searching to expand my competency, but not yet having found a topic of my own. I knew that this was not easy because it had to be something new, in my phantasy something unheard of, but where to find it?
It is always helpful to keep the eyes open and to remain curious. In my case it was the dramatic color change of the so-called purple membrane of Halobacterium salinarum upon lipid extraction. This observation could not be explained by standard chemistry. The rest of the story fits to the dictum of my mentor the Nobel Prize-winning biochemist Feodor Lynen: “Be naive, make an experiment!” And the consequences of this observation and the following experiments became an obsession not only for me and later my lab, but for quite some time a multitude of other laboratories. Now, after more than fifty years, scientific progress with bacteriorhodopsin as a model for membrane proteins reached another peak by the creation of a new field for application of microbial retinal proteins: optogenetics. Therefore, it makes me very happy to share the award with Karl Deisseroth, and the discoverer of channelrhodopsin, Peter Hegemann, my former student.
A large number of colleagues, coworkers and PhD students have contributed to this success, which was not guaranteed at the beginning. On the contrary! In the first years after the discovery of bacteriorhodopsin, the scientific community was very skeptical about the claim of a light-driven proton pump. Skepticism was even stronger towards the claim of bacteriorhodopsin as a second route of photosynthesis in Nature. I remember very vividly the comment of Feodor Lynen when I told him about my idea and the first experiments demonstrating the existence of a proton pump: “I don’t believe it, but I wish you are right!”
Beside the expansion of research into bacteriorhodopsin illuminating its molecular mechanism in ever greater detail, two thematic extensions were essential in my lab: first, the search for more microbial retinal proteins, such as halorhodopsin, as well as a systems biological approach to understand their functional context in the cell. Second, a search for potential applications.
Another development turned out to be a real breakhrough: The discovery of channelrhodopsin by Peter Hegemann and its joint use with halorhodopsin by Karl Deisseroth, who promoted tremendously the new field of optogenetics within the neurosciences.